A long time ago, before the rise of humans, before the first single celled organisms, before the planet even accumulated atmospheric oxygen, Earth was already turning, creating a 24-hour day-night cycle. It’s no surprise, then, that most living things reflect this cycle in their behavior. Certain plants close their leaves at night, others bloom exclusively at certain times of day. Roosters cock-a-doodle-doo every morning, and I’m drowsy by 9:00 pm every night. These behaviors roughly align with the daylight cycles, but internally they are governed by a set of highly conserved molecular circadian rhythms.
Jeffrey Hall, Michael Rosbash and Michael Young were awarded the 2017 Nobel Prize in Physiology/Medicine for their discoveries relating to molecular circadian rhythms. The official statement from the Nobel Committee reads, “…this year’s Nobel laureates isolated a gene that controls the normal daily biological rhythm. They showed that this gene encodes a protein that accumulates in the cell during the night, and is then degraded during the day. [They exposed] the mechanism governing the self-sustaining clockwork inside the cell.” What, then, does this self-sustaining clockwork look like? And how does it affect our daily lives (1)?
This field of research actually dates back into the early eighteenth century when Jean Jacques d’Ortous de Mairan discovered that the mimosa plant, which closes its leaves at night, would continue its cycle even when placed in the dark for days at a time. Later, Erwing Bunning found that circadian rhythms in plants could be inherited, even if the parents were raised in non-circadian light cycles. This led to decades of arguing about the existence of an endogenous circadian clock, as opposed to simple behavioral entrainment. Chronobiology, the study of internal clocks, didn’t thrive as a research community until later in the 1960s (1).
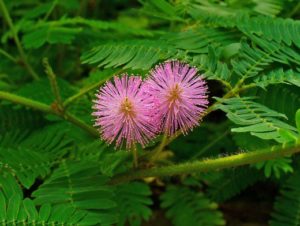
By the 1980s, it was known that mutations in period caused Drosophila flies to have disrupted or absent circadian rhythms, but there was no known molecular mechanism to explain the behavior changes. In 1984, Hall, Rosbash and Young successfully cloned and sequenced the period gene. As they investigated further, they found that the PERIOD (PER) protein cycled in abundance, with its peak during the night. Several years later, the Young lab discovered the timeless gene, which showed the same transcription-translation patterns as period, and that mutating either period or timeless disrupted the cycle of both genes (2–5).
With the foundation of the period gene established, the pieces started coming together to form a picture of a negative feedback loop. The current model of the basic molecular circadian rhythm is called a Transcription-Translation Feedback Loop (TTFL). In the evenings, the two proteins PERIOD and TIMELESS begin to accumulate. These proteins downregulate transcription of their own genes by acting on the transcription factors CLOCK (CLK) and CYCLE (CYC). Protein concentration peaks during the night when transcription of period and timeless has halted and degradation of PER and TIM begins. The protein DOUBLETIME (DBT) is responsible for PER phosphorylation and degradation, and the light-activated CRY protein enhances degradation of TIM. As the protein concentration decreases, transcription increases again, peaking during the day. The timing of this cycle is modulated by post-translational modifications to the proteins, as well as localization and protein complex assembly. One complete cycle takes, as you might have guessed, 24 hours. (6–8).
These mechanisms are clinically relevant for many conditions. One of the most obvious examples is sleep cycles. This molecular clock helps explain why we sleep at night and wake during the day, though problems with the clock can easily disrupt that pattern. Circadian rhythms also govern changes in cortisol levels, blood pressure, body temperature, melatonin secretion. Lifestyles that don’t align with our molecular rhythms are known to lead to neurodegenerative diseases and metabolic disorders, as well as depression and bipolar disorder. This explains some of the long-term dangers of overnight work schedules and chronic night-owl habits (1).
As the days grow shorter in Wisconsin, I won’t always be able to rely on the cycle of daylight to regulate my behaviors. If I did that, I’d never make it to work on time in the mornings. The feedback loop of period and timeless ensures that my body will continue its daily cycles even when the sun sets at 5:30 pm.
References
- Nobel Prize Committee (2017). The 2017 Nobel Prize in Physiology or Medicine – Advanced Information: Discoveries of Molecular Mechanisms Controlling the Circadian Rhythm. Nobelprize.org.
- Bargiello, T.A. et al. (1984). Molecular genetics of a biological clock in Drosophila. Proc Natl Acad Sci, 81. (2142-2146).
- Reddy, P. et al. (1984). Molecular analysis of the period locus in drosophila melanogaster and identification of a transcript involved in biological rhythms. Cell, 38. (701-710).
- Myers, M.P. et al. (1995). Positional cloning and sequence analysis of the Drosophila clock gene, timeless. Science, 270. (805-808).
- Sehgal, A. et al. (1994). Loss of circadian behavioral rhythms and per RNA oscillations in the Drosophila mutant timeless. Science, 263. (1603-1606).
- Darlington, T.K. et al. (1998). Closing the circadian loop: CLOCK-induced transcription of its own inhibitors per and tim. Science, 280. (1599-1603).
- Price, J.L. et al. (1998). Double-timeis a novel Drosophila clock gene that regulates PERIOD protein accumulation. Cell, 94. (83-85).
- Ceriani, M.F. et al. (1999). Light-dependent sequestration of TIMELESS by CRYPTOCHROME. Science, 285. (553-556).
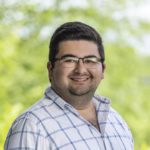
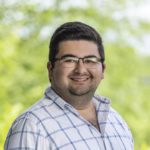
Latest posts by Jordan Villanueva (see all)
- Novel Promega Enzyme Tackles Biggest Challenge in DNA Forensics - November 7, 2024
- Promega Summer Interns Contribute Skills and Ideas from Logistics to R&D - September 10, 2024
- High School Interns Get Hands-On Experience in Promega R&D - September 5, 2024