Today’s blog written by guest author Kendra Hanslik.
In the intricate dance of cellular processes that sustain life, pyruvate emerges as a central figure. It plays a crucial role in the energy production saga. This small molecule is the linchpin between glycolysis and the citric acid cycle, linking the breakdown of glucose to the production of adenosine triphosphate (ATP). In this article, we explore pyruvate’s origins, multifaceted roles, and its association with various diseases.
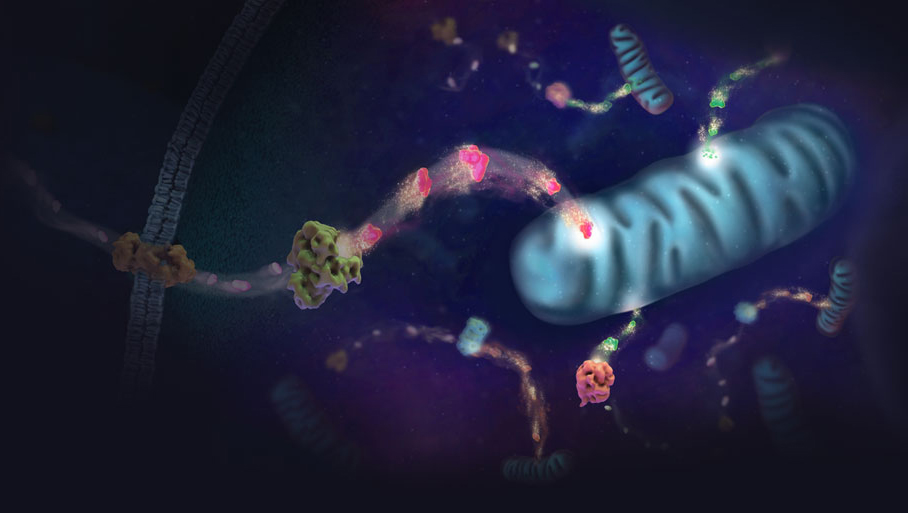
Pyruvate Production
Depending on the environment pyruvate is produced in, this molecule can have many fates. Pyruvate is a three-carbon acid generated as an end-product during glycolysis, the initial stage of glucose metabolism. During the process of glycolysis in the cell’s cytoplasm, glucose is broken down into two pyruvate molecules. This produces a net gain of two ATP and two NADH molecules. At this point, pyruvate is at a crossroads, its destiny determined by the availability of oxygen within the cellular environment.
In the presence of oxygen, pyruvate is transported into the mitochondria where the molecule is converted into acetyl-CoA, initiating the citric acid cycle (TCA cycle). The TCA cycle is a key metabolic hub connecting carbohydrate, fat, and protein metabolism. In the mitochondrial matrix, acetyl-CoA undergoes a series of enzymatic reactions. This generates electron carriers (NADH and FADH2) that pass their high-energy electrons to the electron transport chain. Through oxidative phosphorylation, this will generate most of the ATP produced in cellular respiration.
However, when oxygen is scarce, pyruvate undergoes a reduction reaction that results in ATP production through fermentation. Different organisms employ various fermentation pathways including lactic acid and ethanolic fermentation. These processes replenish NAD+ to sustain glycolysis, enabling cells to continue extracting energy from glucose anaerobically.
Pyruvate’s Versatility: A Building Block for Life
Beyond its role in energy production, pyruvate contributes to the synthesis of essential cell building blocks. It serves as a precursor to produce amino acids, fatty acids, and other biomolecules critical for cell structure and function, thus illustrating that pyruvate functions not only as a fuel source but also as a key player in cellular growth and maintenance. Furthermore, pyruvate can be converted back to glucose through gluconeogenesis, an organism’s mechanism for maintaining blood glucose levels between meals or during fasting. Pyruvate also participates in anaplerotic reactions, which replenishes intermediates in the TCA cycle. Since the TCA cycle is crucial for both energy production and biosynthesis, it is important for the cell to maintain concentrations of TCA cycle metabolites in the mitochondria. Anaplerosis ensures the cycle’s continuous function and underscores pyruvate’s contribution to the metabolic flexibility of cells.
Pyruvate’s Implications in Disease
While pyruvate is a maestro in orchestrating cellular harmony, its dysregulation has been implicated in various diseases. A deficiency in any of the enzymes involved in pyruvate metabolism can lead to many disorders. These disorders can present neurodevelopmental delays, seizures, muscle weakness, and jaundice (Hidalgo et al, 2021; Grace et al, 2018). Aberrant pyruvate metabolism has also been associated with conditions such as cancer and neurodegenerative disorders (Sakamoto et al, 2019; Traxler et al, 2022). For instance, cancer cells often exhibit increased glycolysis despite being in an environment with ample oxygen – this phenomenon is known as the Warburg effect. Pyruvate is known to play a role in this metabolic shift, contributing to the altered cellular energy metabolism observed in cancer cells. Thus, investigating the molecular mechanisms underlying these associations could pave the way for novel therapeutic strategies targeting pyruvate-related pathways.
Conclusion
Pyruvate, often overshadowed by its downstream players such as ATP, is more than a mere intermediary in the journey of glucose metabolism. Its ability to serve as a metabolic chameleon, adapting to the cellular context, highlights its significance in sustaining life. It is a versatile and dynamic player, influencing cellular energy balance, biosynthesis, and even contributing to our understanding of disease mechanisms. As researchers unravel more about the complexities of pyruvate metabolism and the potential for therapeutic interventions, a deeper understanding of cellular physiology continues to unfold. To uncover insights about pyruvate, Promega has developed the Pyruvate-Glo™ Assay, a bioluminescent tool that enables researchers to monitor subtle changes in glycolysis and mitochondrial metabolism by detecting pyruvate levels with a simple two-step protocol that yields results in under 90 minutes. You can learn more details about this specific assay in the technical manual.
This blog is part of a series on cellular energy metabolism, where we explore the importance of various metabolites and their regulation in health and disease. Read other entries about Malate, BHB, BCAA and Glycogen.
References
Grace, R. F., Cohen, J., Egan, S., Wells, T., Witherspoon, B., Ryan, A., Salek, S. S., Bodie, S., & Klaassen, R. J. (2018). The burden of disease in pyruvate kinase deficiency: Patients’ perception of the impact on health-related quality of life. European journal of haematology, 101(6), 758–765. https://doi.org/10.1111/ejh.13128
Hildago, J. Campoverde, L., Ortiz, J.F., Ruxmohan, S., & Eissa-Garcés, A. (2021). A Unique Case of Pyruvate Carboxylase Deficiency. Cureus, 13(5), e15042. https://doi.org/10.7759/cureus.15042
Sakamoto, A., Kunou, S., Shimada, K., Tsunoda, M., Aoki, T., Iriyama, C., Tomita, A., Nakamura, S., Hayakawa, F., & Kiyoi, H. (2019). Pyruvate secreted from patient-derived cancer-associated fibroblasts supports survival of primary lymphoma cells. Cancer science, 110(1), 269–278. https://doi.org/10.1111/cas.13873
Traxler, L., Herdy, J. R., Stefanoni, D., Eichhorner, S., Pelucchi, S., Szücs, A., Santagostino, A., Kim, Y., Agarwal, R. K., Schlachetzki, J. C. M., Glass, C. K., Lagerwall, J., Galasko, D., Gage, F. H., D’Alessandro, A., & Mertens, J. (2022). Warburg-like metabolic transformation underlies neuronal degeneration in sporadic Alzheimer’s disease. Cell metabolism, 34(9), 1248–1263.e6. https://doi.org/10.1016/j.cmet.2022.07.014
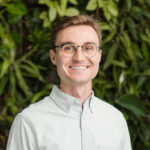
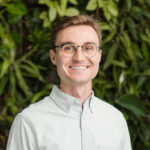
Latest posts by Simon Moe (see all)
- Alzheimer Disease and Metabolic Dysfunction: A Critical Intersection in Brain Health - February 26, 2025
- Glo-ing Above and Beyond: Simplifying Science with MyGlo Reagent Reader - January 27, 2025
- The Greatness of Glycogen: A Central Storage Molecule in Energy Metabolism - January 3, 2025