Today’s blog written by guest author Kim Haupt.
Cellular energy metabolism is a complex biological process that relies on a suite of metabolites, each with distinct roles to maintain. Malate is one of these metabolites and is essential for maintaining cellular function through important roles in both energy production and redox homeostasis. In this blog, we highlight malate’s diverse roles and uncover some of its connections to human disease.
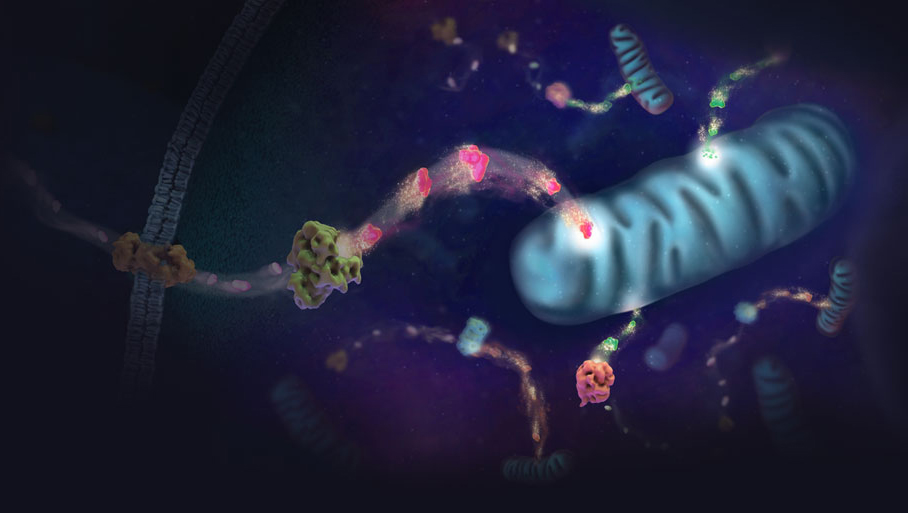
Malate’s Versatile Roles in Metabolism
Malate is an intermediate in the TCA cycle (also called the citric acid cycle or the Krebs cycle), which is the central mitochondrial respiration pathway for production of ATP. During the TCA cycle, fumarase catalyzes the reversible hydration of fumarate to malate. This reaction is essential for the continued function of the TCA cycle and energy production in cells.
Malate plays a critical role in maintaining the redox balance, which is the equilibrium between oxidation and reduction reactions in a biological system. This maintenance occurs through the malate-aspartate shuttle. The malate-aspartate shuttle transfers NADH from the cytosol into the mitochondria where it is utilized in the electron transport chain to produce ATP. This process ensures the maximum yield of ATP from glucose metabolism and maintains the necessary redox balance for continued cytosolic glycolytic activity.
Beyond its roles in energy production, malate contributes to the synthesis of amino acids and other essential biomolecules, supporting cellular growth and maintenance. During periods of high energy needs, malate can serve as an anaplerotic substrate to replenish depleted TCA cycle intermediates. This maintenance ensures the cycle’s continuous function and efficient ATP production. Appropriate malate levels and function are necessary for both metabolic flexibility and homeostasis.
Malate and Implications for Disease
Dysregulation of malate metabolism has been linked to various diseases, especially cancer. In hypoxic tumor environments, the TCA cycle can run in reverse to maintain proliferation and support lipogenesis. Malate serves as a key metabolite in this reversed cycle, contributing to the production of acetyl CoA, citrate, and fatty acids, which are essential for cancer cell growth and survival (Filipp, et al 2012). Malate can also be converted to pyruvate by malic enzyme, generating NADPH in the process; NADPH is critical in cancer cells to support anabolic reactions and managing oxidative stress (Ju, et al 2022). Consistent with these observations, malic enzyme and malate dehydrogenase overexpression in tumors is associated with cancer growth and metastasis, making these enzymes potential targets for therapeutic intervention (Faubert, et al 2020).
There are also several rare, genetically inherited disorders that affect metabolism of this specific metabolite. Most of these involve dysfunction of enzymes in the TCA cycle or the malate-aspartate shuttle, and result in a range of metabolic and neurological symptoms (Broeks, et al 2021; Ryder, et al 2018, Shamim et al 2021). For example, genetic alterations of the malate aspartate shuttle typically result in consistent infantile epileptic encephalopathy, or epileptic seizures. (Broeks, et al 2021).
The link between malate and various diseases suggests potential therapeutic targets in pathways that specifically modulate this metabolite. Monitoring the abundance of malate in both healthy and diseased tissues can reveal mitochondrial metabolism dysfunction and redox imbalances. These insights may lead to new approaches for disease treatment and management.
Conclusion
Malate is a versatile player in cellular energy homeostasis. Research will continue to reveal the intricacies of malate metabolism and its implications for health and disease. To facilitate new discoveries, Promega has developed the Malate-Glo™ Assay. This assay features a simple two-step protocol, delivering results in under 90 minutes. It allows researchers to detect subtle changes in malate levels, TCA cycle activity, and mitochondrial metabolism. For more details, refer to the technical manual.
This blog is part of a series on cellular energy metabolism, where we explore the importance of various metabolites and their regulation in health and disease. Read other entries about Pyruvate, BHB, BCAA and Glycogen.
References
Filipp, F. V., Scott, D. A., Ronai, Z. A., Osterman, A. L., & Smith, J. W. (2012). Reverse TCA cycle flux through isocitrate dehydrogenases 1 and 2 is required for lipogenesis in hypoxic melanoma cells. Pigment Cell & Melanoma Research, 25(3), 375-383. https://doi.org/10.1111/j.1755-148X.2012.00989.x
Ju, H. Q., Lin, J. F., Tian, T., Xie, D., & Xu, R. H. (2020). NADPH homeostasis in cancer: functions, mechanisms and therapeutic implications. Signal Transduction and Targeted Therapy, 5(1), 231. https://doi.org/10.1038/s41392-020-00326-0
Faubert, B., Solmonson, A., & DeBerardinis, R. J. (2020). Metabolic reprogramming and cancer progression. Science, 368(6487), eaaw5473. https://doi.org/10.1126/science.aaw5473
Broeks, M. H., van Karnebeek, C. D. M., Wanders, R. J. A., Jans, J. J. M., & Verhoeven-Duif, N. M. (2021). Inborn disorders of the malate aspartate shuttle. Journal of Inherited Metabolic Disease, 44(5), 1029-1045. https://doi.org/10.1002/jimd.12402
Ryder, B., Moore, F., Mitchell, A., Thompson, S., Christodoulou, J., & Balasubramaniam, S. (2018). Fumarase Deficiency: A Safe and Potentially Disease Modifying Effect of High Fat/Low Carbohydrate Diet. JIMD Reports, 43, 131-137. https://doi.org/10.1007/8904_2018_120
Shamim, A., Mahmood, T., Ahsan, F., Kumar, A., & Bagga, P. (2021). Genetics of Alzheimer’s Disease: Review of Novel Mutations. Journal of Molecular Neuroscience, 71(9), 1741-1755. https://doi.org/10.1007/s12031-021-01850-6
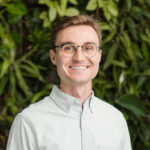
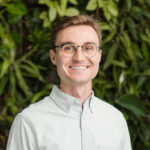
Latest posts by Simon Moe (see all)
- Alzheimer Disease and Metabolic Dysfunction: A Critical Intersection in Brain Health - February 26, 2025
- Glo-ing Above and Beyond: Simplifying Science with MyGlo Reagent Reader - January 27, 2025
- The Greatness of Glycogen: A Central Storage Molecule in Energy Metabolism - January 3, 2025