Approximately 30 million years ago, a retrovirus integrated into the germline of a common ancestor of baboons, gorillas, chimpanzees and humans. That endogenous retrovirus, now known as gammaretrovirus human endogenous retrovirus 1 (HERV-1), may provide clues about the aberrant regulation of gene transcription that enables tumor cells to grow and survive.
Understanding the Mechanism Behind Cancer Gene Expression
Scientists have long described the striking differences in gene expression, signaling activity and metabolism between cancer cells and normal cells, but the underlying mechanisms that cause these differences are not fully understood. In a recent Science Advances article, published by Ivancevic et al., researchers from the University of Colorado, Boulder; the University of Colorado Anschutz Medical Campus, and the University of Colorado School of Medicine report their efforts to identify endogenous retrovirus elements that might be part of the answer to the complex question of what biological events are responsible for the changes in gene expression in cancer cells.
The researchers hypothesized that transposable elements (TEs), specifically those associated with endogenous retroviruses could be involved in cancer-specific gene regulation. Endogenous retroviruses (ERVs) are the remnants of ancient retroviral infections that have integrated into the germline of the host.
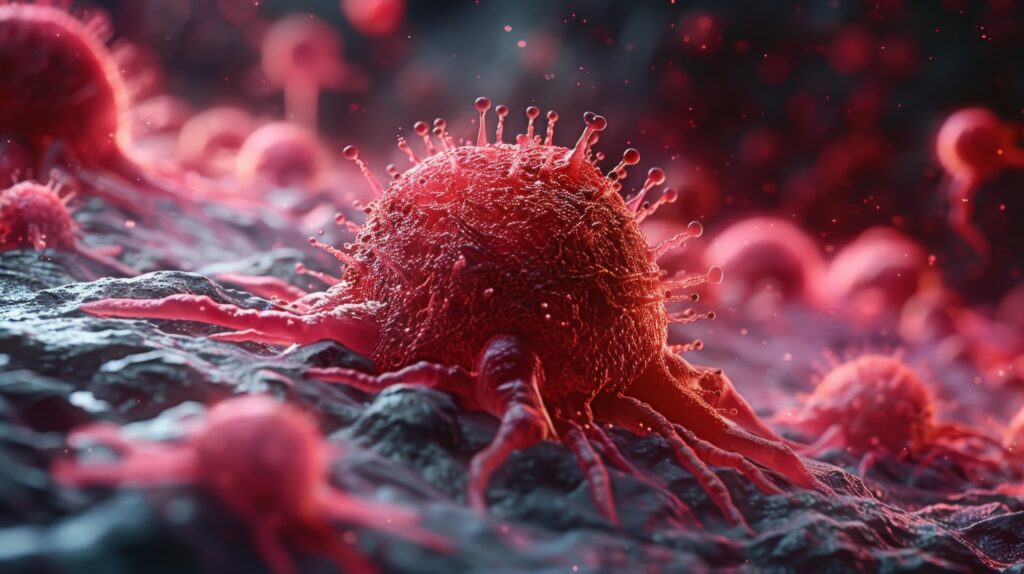