Mitogen-activated protein kinases (MAPKs) are a large family of proteins that regulate diverse cellular functions in eukaryotes, including gene expression, proliferation, differentiation and apoptosis (1). MAPK signaling pathways typically include three sequentially activated kinases, and these pathways are triggered in response to extracellular stimuli, such as cytokines, mitogens, growth factors and oxidative stress (1). Ultimately, the signal is transmitted to the nucleus, with the activation of a specific transcription factor that modulates the expression of one or more genes.
Among MAPK pathways, the RAS-RAF-MEK-ERK signaling pathway has been studied extensively. Mutations in RAS family proteins and resultant dysregulation of the signaling pathway are implicated in a variety of cancers. Therefore, this pathway is a popular target for anticancer drug development.
However, drug discovery and development researchers looking to target the RAS family face a significant challenge. RAS proteins are small GTPases that bind GTP with high affinity, and they possess few accessible sites that can be targeted by small-molecule inhibitors. Therefore, RAS has historically been considered an undruggable target (2). Although new approaches—such as using targeted protein degradation to destroy RAS isoforms—show some promise, most drug discovery efforts have focused on downstream members of the signaling pathway. Since RAF acts immediately downstream from RAS, considerable attention has been devoted to finding effective small-molecule RAF inhibitors.
RAF Dimerization and Signaling
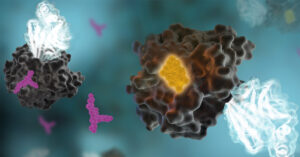
Three RAF isoforms are known in mammals, originating from three independent genes: ARAF, BRAF and CRAF. BRAF mutations are common oncogenic drivers that either directly or indirectly activate the RAS-RAF signaling complex, and BRAF has been studied extensively (3). RAF isoforms contain three highly conserved regions known as CR1, CR2 and CR3 (4). CR1 contains a primary and a secondary RAS-binding domain. The primary domain interacts with active, GTP-bound RAS. The secondary, cysteine-rich domain is required for the interaction of CR1 and the kinase domain in CR3 (3).
The CR2 region interacts with a family of regulatory proteins, known as 14-3-3, to form inactive RAF dimers in a “closed” conformation (3). Binding of activated RAS, followed by several phosphorylation and dephosphorylation events, results in a dimerized, highly active “open” RAF conformation. Although all RAF protomers can form homodimers as well as heterodimers, BRAF:CRAF heterodimers are the most effective in activating MEK (5).
Most drug discovery efforts have focused on BRAF variants due to their oncogenic role in a variety of human cancers. Several BRAF-selective kinase inhibitors are effective in BRAF mutant cancer cells, but they do not block ERK activation in RAS mutant cells (6). Further, these first-generation inhibitors promote RAS-dependent RAF dimerization in RAS mutant cells and, ultimately, can lead to activation of the RAF-MEK-ERK pathway—the so-called “RAF inhibitor paradox” (5, 6).
Breaking the Paradox
Consequently, alternative drug development strategies have emerged to avoid the RAF inhibitor paradox (5). “Pan-RAF inhibitors” target both active RAF dimers and monomers, locking RAF proteins into conformations that induce RAF dimerization without activating ERK signaling. “Paradox breakers” bind BRAF monomers, block dimerization, and do not activate ERK signaling.
These drug development strategies rely on sensitive, accurate methods to measure drug-target occupancy within active RAS-RAF complexes in live cells. A recent collaboration between scientists at Promega and Vibliome Therapeutics aimed to develop such a method in HEK293 cells (7), based on NanoBRET™ target engagement technology.
“Understanding how small-molecule RAF inhibitors bind to all three RAF paralogs is critical for the development of next-generation drugs against this family,” says Aaron Crapster, PhD, Vice-President of Biology at Vibliome, and a co-corresponding author on the publication. “There is interest in pan-RAF inhibitors as well as paralog-selective inhibitors (e.g., CRAF-selective inhibitors).”
The method uses a conditional BRET reporter system based on an energy transfer donor originating from NanoLuc®-RAF protomer fusions. Cell-permeable, ATP-competitive fluorescent probes measure engagement at the luminescent complex in live cells expressing endogenous KRAS (low RAS) or untagged KRAS mutants (high RAS). Thus, the method enables quantitative, protomer-selective readouts for BRAF, CRAF or ARAF in the KRAS-RAF complex. Using this approach, the study reported on the relative selectivity of RAF dimer inhibitors for BRAF/CRAF over BRAF/ARAF heterodimers in the presence of hotspot mutant alleles of KRAS (7).
A key finding was that only engagement of ARAF was commensurate with inhibition of MAPK signaling. “Clearly, research is uncovering a previously overlooked role for ARAF in KRAS signal transduction,” Dr. Crapster says. The results have implications for targeting RAF to treat KRAS mutant–driven (and, potentially, certain BRAF mutant–driven) cancers. Since many druggable kinases propagate signals via oligomeric complexes, the methods described in this publication hold promise for broader applications. “The ability to specifically probe the pharmacology of small-molecule kinase inhibitors within the context of a defined cellular system should enable the development of more effective drugs,” Dr. Crapster concludes.
Learn more about using NanoBRET™ Target Engagement technology to measure target affinity and occupancy at RAF kinases in dimeric complexes.
References
- Johnson, G.L. and Lapadat, R. (2002) Mitogen-activated protein kinase pathways mediated by ERK, JNK, and p38 protein kinases. Science 298, 1911–1934.
- Fernández-Medarde, A. et al. (2021) 40 years of RAS—a historic overview. Genes 12, 681.
- Matallanas, D. et al. (2011) Raf family kinases: old dogs have learned new tricks. Genes Cancer 2(3), 232–260.
- Zhao, J. and Luo, Z. (2022) Discovery of Raf family is a milestone in deciphering the Ras-mediated intracellular signaling pathway. J. Mol. Sci. 23, 5158.
- Cook, F.A. and Cook, S.J. (2020) Inhibition of RAF dimers: it takes two to tango. Biochem. Soc. Trans. 49, 237–251.
- Cox, A.D. and Der, C.J. (2010) The Raf inhibitor paradox: unexpected consequences of targeted drugs. Cancer Cell 17, 221–223.
- Vasta, J.D. et al. (2023) Protomer selectivity of type II RAF inhibitors within the RAS/RAF complex. Cell Chem. Biol. 30, 1–12.
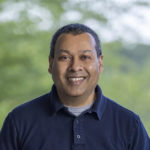
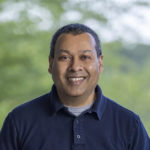
Latest posts by Ken Doyle (see all)
- Will Artificial Intelligence (AI) Transform the Future of Life Science Research? - February 1, 2024
- RAF Inhibitors: Quantifying Drug-Target Occupancy at Active RAS-RAF Complexes in Live Cells - September 5, 2023
- Synthetic Biology: Minimal Cell, Maximal Opportunity - July 25, 2023