What is the Developmental Clock?
The development of the human embryo is a complicated process that involves careful coordination of thousands of genes. Just like musical instruments in an orchestra, each gene performs its role—sometimes silent, sometimes intense—but always right on cue. The tempo of the symphony, or the speed of embryonic development, depends on an intrinsic biological clock known as the developmental clock. The developmental clock is like the conductor of the orchestra, controlling the tempo of the music and ensuring that each gene is expressed at the right moment with the right intensity. If just one gene is expressed too soon or going one beat too fast, it could disrupt the harmony of the whole symphony, resulting in an improperly developed embryo.
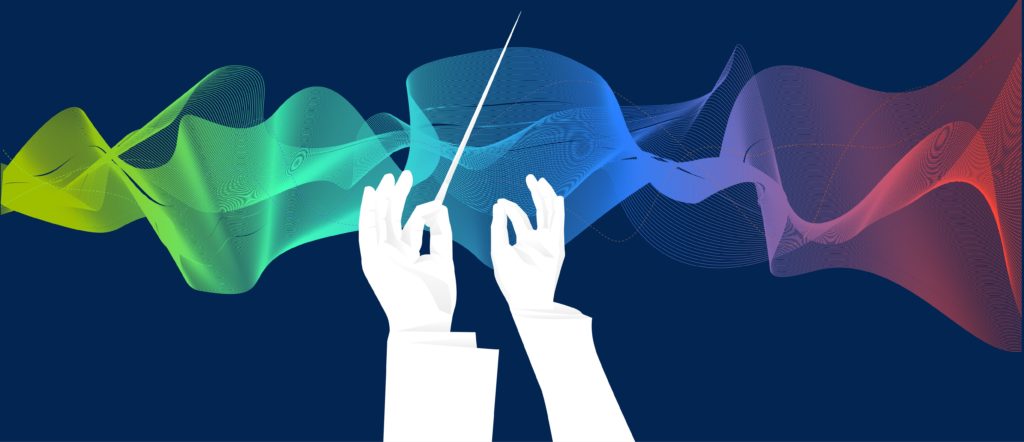
One example of what could happen when the developmental clock is disrupted is a disease called spondylocostal dysostosis (SCDO). SCDO is a genetic disorder that causes abnormal formation of the spine and ribs. Patients often have a short neck and trunk, and an abnormal curvature in the spine (scoliosis). SCDO can be caused by a mutation in the HES7 gene. HES7 is an “oscillating gene”, a kind of gene that is expressed in a rhythmic pattern—like the beating of a drum. This rhythm is essential for forming our ribs and each vertebra of our spine—a process known as “segmentation”—during early embryonic development.
Understanding the Human Segmentation Clock
The segmentation process is controlled by a specific developmental clock, called the “segmentation clock.” Our vertebrate columns are evenly spaced because there is a very specific length of time in which HES7 levels repeatedly oscillates up and down in a cycle, which corresponds to each pair of vertebrae forming. The segmentation clock has been studied in many different species, and the tempo of this clock differs among them. In mice, the cycle happens every 2 hours; in chickens, it’s every 1.5 hours; in zebrafish, it’s every 0.5 hour. However, the segmentation clock in humans is largely a mystery, because it is difficult to access such early-stage embryos due to ethical and technical issues.
Dr. Li-Fang Chu, Assistant Professor at the University of Calgary, is interested in studying the developmental clock and how it is regulated. “The developmental clock is extremely elegant, and to this day we still don’t know how it happens. It’s a very elaborate system that is a daunting task to understand,” says Dr. Chu, “You can’t use other model organisms to explain how human segmentation works. We have to use human cells. If we can capture it in human cells, it will be a stepping stone to further understand how the human developmental clock is controlled.”
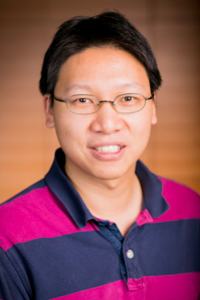
Bioluminescence Live-Cell Imaging
In 2019, Dr. Chu succeeded in creating a human embryonic stem cell (ESC) model in a lab dish that replicates the genetic profiles of the human segmentation clock. Using an optimized differentiation protocol, he directed the humans ESCs towards a cell state in which segmentation occurs. To observe the expression of oscillating genes in real-time, he used bioluminescence live-cell imaging—a method that allowed him to visualize gene expression levels based on light intensity.
First, he performed gene editing to tag HES7 with NanoLuc® luciferase, a modified version of the luciferase found in bioluminescent deep sea shrimp. This allowed him to observe how much HES7 is being expressed based on luminescent signal: the brighter the light, the more HES7 in the cells. Then, he added a formulation of the Nano-Glo® Vivazine™ Live Cell Substrate, which is required for the luciferase reaction to occur, and continuously detected luminescence every 5 minutes for 32 hours.
“We detected gene oscillation activity almost from the very first experiment, which is a huge surprise,” he says. “They are just a pile of cells in a plastic dish. Why would they display this kind of dynamic in a matter of hours?” But he found that the gene oscillation patterns that occurred in the dish were extremely precise—they behaved just like a clock.
Using a luminescent microscope, he was able to see a consistent flash of light, indicating a rise in HES7 expression, every 5 hours. This bioluminescence live-cell imaging experiment was the first time the exact timing of oscillating genes in early human development has been observed.
Dr. Chu believes that the key to the success of his project was the ability to collect data in real time with high temporal resolution. “In the cell, things are very dynamic; they are happening every second. If we hadn’t been able to collect data every 5 minutes, we would have completely missed this clock. The bioluminescence live-cell imaging was what allowed us to discover this dynamic,” he says.
Future Clinical Applications
Reproducing the developmental clock in cells has many clinical implications. For one, it provides a cell model to study diseases caused by early defects in the developmental clock, such as SCDO. It may also be useful for producing mature stem cells for clinical therapies. A huge obstacle in stem cell therapy is that it takes a long time for human stem cells to differentiate. In most cases, cells have to be differentiated for months before they are mature enough to use in a clinical setting. What if we could speed up that process?
According to Dr. Chu, controlling the developmental clock could be possible, and the key may be comparing the human clock with those of different mammalian species. If they could figure out what causes the human clock to go slower than other species, they could manipulate that mechanism to speed things up in human cells. This could drastically reduce the time and effort required to produce full organs for transplants in the future.
Of course, that is a long way ahead. Dr. Chu is careful to note that although they have succeeded in mimicking the developmental clock in human ESCs, they are still “just a pile of cells” and are far from forming any relevant tissue or organ. Nonetheless, this is an important first step that could provide a window into understanding how we develop in the earliest stages of our lives.
Reference: Chu, L.F. et al. (2019) An In Vitro Human Segmentation Clock Model Derived from Embryonic Stem Cells. Cell Rep. 28(9): 2247–55
Learn more about bioluminescence live-cell imaging at our website.
To learn more about the last 30 years of bioluminescent innovations and the discoveries they’ve enabled, please visit our 30th anniversary celebration page.
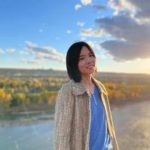
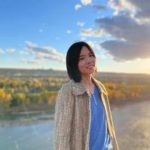
Latest posts by Johanna Lee (see all)
- A Diabetes Drug, Metformin, Slows Aging in Male Monkeys - December 19, 2024
- Understanding Persistent Digestive Disorders With Multiplex PCR - August 22, 2024
- Can AI Replace High-Throughput Screens for Drug Discovery? - April 30, 2024