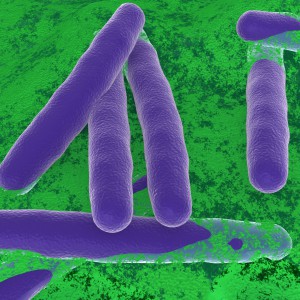
Microbial cells outnumber the cells of our own bodies approximately 10:1, these microbes that live on our skin and along the epithelial linings of our internal tubes make up our microbiota*, and they can have major effects on our health. Most of our microbiota are commensal organisms, living in harmony with our body, but if you suppress our immune system or greatly reduce their populations with large doses of antibiotics, and you will soon see the effects of disrupting our microbiota.
There is much interest in the microbiota that inhabit our bodies. For instance several studies have indicated that intestinal microbes can play a big part in obesity, with changes in the makeup of the microbiota being a major risk factor (1). But many of these organisms are hard to learn about—the ones that inhabit the deep folds of our gut thrive in moist, warm, anaerobic conditions with lots of specialized nutrients, conditions that are very hard to replicate in the laboratory. For that reason, we don’t know much about many of the microbes that are the most abundant within us.
The Human Microbiome Project begun in 2008 by the National Institutes of Health (2) seeks to understand human microbiota and their relationship to human health. To do this, the researchers leading the project took a metagenomic approach—using advanced DNA sequencing technologies to sequence the genomes of human microbiota and get a look at the human microbiome—without culturing the microbes.
But to truly understand their biology, and to perhaps exploit what we learn to enhance human health we need to be able to manipulate these organisms. In particular, biologists who are interested in synthetic biology would like to use these micro-organisms to monitor what is going on in our bodies, particularly our guts. What better monitor for these hard-to-access places than an organism that is already well adapted to live there?
Synthetic genetic circuits are a way of manipulating genetics to engineer bacteria or viruses to act as sensors to record environmental or cellular signals or accomplish a particular task in a biological system. Well studied micro-organisms have already been programmed to accomplish specific tasks. In 2003 scientists reported engineering attenuated strains of Salmonella typhimurium to express an E. coli gene capable of converting 5-fluorocytosine to 5-fluorouracil (5-FU) to treat cancers. Because the S. typhimurium tend to colonize the anaerobic, fast growing tumor environments the 5-FU conversion occurred at those sites (3). Listeria monocytogenes has been used a vector for delivering human interleukin 10 to treat inflammatory bowel disease (4).
In a paper published in July 2015, Mimee et al. (4) describe work with Bacteriodes thetaiotaomicron, a commensal bacterium of the human gut that is present in 46% of the human population, is highly stable and abundant. That stability and abundance makes it a good choice for long-term studies of the gut environment and the metabolic events occurring there—if genetic circuits can be created that can translate cellular and environmental signals into long-term cellular memory.
First, Mimee and his colleagues constructed constitutive circuits—circuits that would permanently activate a gene in response to a signal. They used a variety of combinations of the promoter for the housekeeping sigma factor from B. thetaiotaomicron, ribosome binding sites (RBSs) of varying strengths, and the NanoLuc® luciferase reporter to monitor gene expression. Their goal was to create genetic circuits allow modulation of gene expression over several orders of magnitude. From the literature and their own library constructions, they were able to identify 8 RBSs that spanned a 103-fold expression ranges. Combinations of the promoters and RBSs produced a 104-fold expression range, giving researchers a range of gene expression that is comparable to more well studied laboratory organisms.
After establishing that they could create constitutive circuits, Mimee and colleagues next set out to create inducible circuits: The target gene (in this case the NanoLuc® Luciferase reporter) would only be activated in the presence of a particular signal. The Luciferase gene was placed under the control of the rhamnose promoter such that luciferase expression was only activated in the presence of the sugar rhamnose. They added “cellular memory” to the inducible circuit by incorporating integrases under the control of a hramnose promoter and integrase recognition sites flanking a unique DNA sequence. In the presence of rhamnose, luciferase expression increased and the circuit also produced integrase, which clipped out the unique piece DNA from the circuit, flipped it around and replaced it—creating a permanent record in the DNA of the encounter with the signal.
Finally, they worked on creating circuits that could inactivate genes or otherwise modify the genetic circuit by adding CRISPR interference (gene silencing) technology to their scheme. The CRISPR/Cas9 System was originally found in bacteria and seems to function normally as an adaptive immune system against viral infections. But, to the genetic engineer it’s a handy way of creating breaks in DNA at specific places. The CRISPRi technology needs two components, a guide RNA (responsible for the specificity of the break) and the Cas9 endonuclease that actually creates the break in the DNA. Several forms of Cas9 exist, including ones that only nick a single strand of DNA and ones that only bind the DNA, preventing other molecules like activators from accessing it.
Using this system they engineered circuits repressed gene expression by activating Cas9 activity when a specific signal was received. They designed their CRISPRi system to knockdown NanoLuc luciferase expression in synthetic constructs, then expanded it to use additional guide RNAs knockdown endogenous genes.
To be useful as a sensor, the engineered B. thetaiotaomicron must be maintained in the gut and compete successfully with the other microbes that inhabit it. They were able to successfully colonize mouse gut with their engineered cells, demonstrating successful colonization both by qPCR analysis of DNA isolated from stool samples and luciferase assays of stool samples. Furthermore the genetic circuits retained their functions as sensors of the gut environment.
In this study, the authors published evidence of viable synthetic genetic circuits that allow manipulation of the stable, abundant human commensal bacterial species B. thetaiotaomicron. This new ability to manipulate an established resident of the human gut to act as a long-term sensor will eventually enable long-term analysis of how changes in resident microflora, metabolic events and the gut environment are linked to health and disease.
*Author’s note: I am using microbiota to refer to the collection of microbial organisms and microbiome to refer to their collective genomes in this article.
References
- Ley, R.E. (2010) Obesity and the human microbiome Curr. Opin. Gastroenterology 26, 5–11.
- NIH Human Microbiome Project Web Site http://hmpdacc.org/overview/about.php (accessed 8/13/2015)
- Nemunaitis, J. et al. (2003) Pilot trial genetically modified, attenuated Salmonella expressing the E. coli cytosine deaminase gene in refractory cancer patients. Cancer Gene Ther. 10, 737–44.
- Steidler, L. et al. (2003) Biological containment of genetically modified Lactoccocus lactis for intestinal delivery of human interleukin 10. Nature Biotechnology 21, 785–89.
- Mimme, M. et al. (2015) Programming a human commensal bacterium, Bacteroides thetaiotamicron, to sense and respond to stimuli in the murine gut micro biota. Cell Systems 1, 1–10.
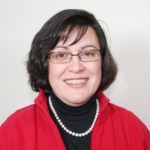
Michele Arduengo
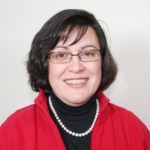
Latest posts by Michele Arduengo (see all)
- Unlocking the Secrets of ADP-Ribosylation with Arg-C Ultra Protease, a Key Enzyme for Studying Ester-Linked Protein Modifications - November 13, 2024
- Exploring the Respiratory Virus Landscape: Pre-Pandemic Data and Pandemic Preparedness - October 29, 2024
- From Fins to Genes: DNA Barcoding Unlocks Marine Diversity Along Mozambique’s Coast - October 15, 2024