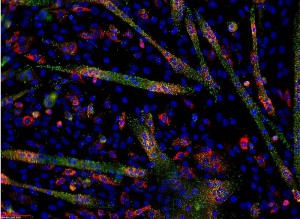
Some of us scientists who have been around for a while still think about RNA molecules falling into three categories: messenger RNA (mRNA), ribosomal RNA (rRNA) and transfer RNA (tRNA). However, I have revised my outdated RNA classification scheme as scientists discover exciting new classes of RNAs that do some fairly amazing things. For example, in the early 1980s, Thomas Cech discovered ribozymes, RNAs that have catalytic functions (1), and in the early 1990s, researchers began to take interest in short noncoding RNAs that act as a genetic regulators, the first of which was discovered in C. elegans (2). RNA is no longer simply a biological middleman between DNA and protein. These ephemeral nucleic acid molecules play a much bigger role of cellular physiology and gene regulation than we had previously ascribed to RNA.
Consider the microRNA. At only about 21–26 nucleotides in length, microRNAs (miRNAs) are short, hence the name. You may be tempted to dismiss miRNAs as too short to accomplish much of anything, but don’t be fooled; these RNA molecules are a multifunctional workhorse. miRNAs play a key role in a number of genetic regulatory mechanisms throughout the plant and animal kingdoms and even in certain viruses. Scientists estimate that the human genome encodes about 2,000 different miRNAs and miRNAs account for about 3–4% of human genes (3). Only the Y chromosome seems bereft of these regulatory genes.
There are many mechanisms by which miRNAs can silence a gene (reviewed in references 3 and 4), and in some cases, several miRNAs can bind to the same target mRNA and cooperatively regulate gene expression for more precise control. While the miRNA binds directly to its sequence-specific target sites, repression often is indirect, mediated by other factors or enzymes that are recruited to form effector complexes called microribonucleoproteins (miRNPs). These miRNPs can act at many different stages of gene expression.
MicroRNAs can have a regulatory effect even before transcription occurs. miRNAs play a role in epigenetic gene regulation (reviewed in references 5 and 6) and can direct both cytosine methylation and histone modification to silence DNA repeats in the genome. For example, a class of 29-nucleotide RNAs that was first discovered through their interaction with the spermatogenesis-specific PIWI protein (piwi-interacting RNAs; piRNAs) map to repetitive DNA sequences and are important for silencing short interspersed elements (SINEs), long interspersed elements (LINEs) and long terminal repeat (LTR) retrotransposons.
miRNAs also can affect mRNA stability. Annealing of an miRNA to a complementary mRNA and formation of an miRNP can result in mRNA endolytic cleavage or destabilization and degradation through shortening of the poly(A) tail and accelerated mRNA decapping. miRNAs also can interfere with translation, often by binding to the 5´ or 3´ untranslated region (UTR). miRNAs can recruit proteins that interfere with cap-binding proteins and translation initiation factors, resulting in reduced translation initiation. miRNAs also can wreak havoc by recruiting other miRNP components that obstruct ribosome binding or assembly, promote ribosome dissociation, compete with translation elongation factors or even target degradation of the resulting polypeptide.
Although the regulatory mechanisms mentioned above result in gene repression, there is increasing evidence to support the idea that miRNAs also can stimulate gene expression, both directly and indirectly (reviewed in reference 7).
Expression of individual miRNP components and cofactors can vary depending on cell type, developmental stage and even environmental conditions, leading to another layer of regulatory complexity. This allows cells to regulate genes quickly and precisely.
All this regulatory power from a short little RNA molecule. There should be no wonder why microRNAs are such a big deal.
References
- Kruger, K. et al. (1982) Self-splicing RNA: Autoexcision and autocyclization of the ribosomal RNA intervening sequence of Tetrahymena. Cell 31, 147–57. http://pmid.us/6297745
- Lee, R.C. et al. (1993). The C. elegans heterochronic gene lin-4 encodes small RNAs with antisense complementarity to lin-14. Cell 75, 843–54. http://pmid.us/8252621
- Valinezhad Orang, A., Safaralizadeh, R. and Kazemzadeh-Bavili, M. (2014) Mechanisms of miRNA-mediated gene regulation from common downregulation to mRNA-specific upregulation. Int. J. Genomics Article ID 970607 http://dx.doi.org/10.1155/2014/970607
- Wilczynska, A. and Bushell, M. (2015) The complexity of miRNA-mediated repression. Cell Death Differentiation 22, 22–33. http://www.nature.com/cdd/journal/v22/n1/full/cdd2014112a.html
- Sato, F. et al. (2011) MicroRNAs and epigenetics. FEBS J. 278, 1598–1609. http://onlinelibrary.wiley.com/doi/10.1111/j.1742-4658.2011.08089.x/full
- Costa, F.F. (2008) Non-coding RNAs, epigenetics and complexity. Gene 410, 9–17. http://pmid.us/18226475
- Vasudevan, S. (2012) Posttranscriptional upregulation by microRNAs, Wiley Interdisciplinary Reviews: RNA 3, 311–30. http://pmid.us/22072587
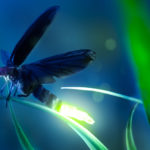
Terri Sundquist
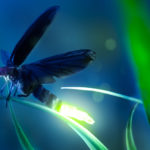