In late November 2023, regulatory authorities in Japan approved a new SARS-CoV-2 vaccine. Unlike earlier messenger RNA (mRNA) vaccines used to protect against COVID-19, this one relies on a technology called self-amplifying mRNA, or saRNA. Though researchers have long pursued saRNA-based vaccines, this represents the first full approval for the technology in humans and marks an exciting advance in the ongoing development of mRNA vaccines.
Continue reading for an overview of how saRNA vaccines work and some of their advantages relative to standard mRNA vaccines.
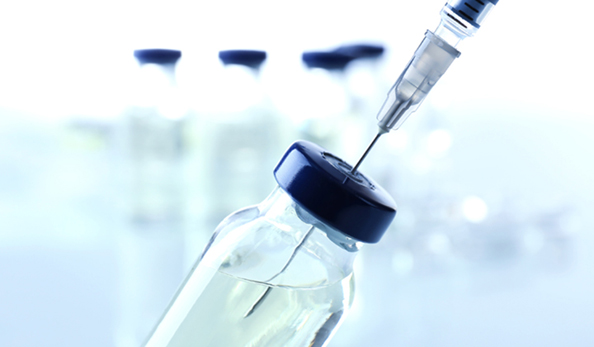
Understanding saRNA Vaccines
mRNA vaccines deliver genetic information to host cells that enable them to translate vaccine mRNA into antigenic viral proteins, such as the SARS-CoV-2 spike protein. Self-replicating mRNA, also known as replicons, function similarly but also contain replicase genes that encode an RNA polymerase complex, which can amplify the vaccine mRNA (1).
As science journalist Elie Dolgin explains in a Nature news article, it’s like having a built-in printing press that produces additional vaccine in cells.
The addition of replicase genes can therefore increase the amount of vaccine mRNA in cells, which likewise yields increased expression of the protein of interest relative to the initial vaccine dose. In theory, this means saRNA vaccines could produce the necessary antigenic proteins of interest required for an immune response at lower doses than standard mRNA vaccines (1).
saRNA in the Clinic
ARCT-154 (or VBC-COV19-154) received approval from Japan’s Ministry of Health, Labour and Welfare as a COVID-19 vaccine and booster for adults. In December 2023, a peer-reviewed article was published summarizing clinical trial results where ARCT-154 or a standard mRNA vaccine were administered as a fourth-dose booster (2). The trial involved 828 adult participants and the resulting immune response was measured 28 days after the booster dose.
- The booster dose was “equally well tolerated” by the study participants.
- A booster dose of ARCT-154 produced comparable levels of SARS-CoV-2 neutralizing antibodies for the original SARS-CoV-2 strain compared to a booster dose of a mRNA COVID-19 vaccine.
- A booster dose of ARCT-154 produced higher levels of SARS-CoV-2 neutralizing antibodies for the Omicron BA.4/5 variant compared to a booster dose of a mRNA COVID-19 vaccine.
Although this is the first saRNA vaccine to receive full approval for use in humans, another COVID-19 saRNA vaccine received an emergency use authorization in India in 2022. Other clinical trials of COVID-19 vaccines based on saRNA vaccines have been initiated (3). Additionally, saRNA vaccines are also an area of interest for use in veterinary applications (4).
Potential Benefits
saRNA vaccines share many of the same benefits of mRNA vaccines. A major one is that they can be produced via cell-free manufacturing methods that are faster and less cumbersome than traditional cell-based culture methods (1). So, in theory, both mRNA and saRNA vaccines can be rapidly designed and manufactured to address emerging pathogens.
Compared to mRNA vaccines the advantage saRNA vaccines offer is the opportunity to use lower vaccine doses. In the lab, lower doses of saRNA vaccines have been shown to yield comparable levels of immune protection as standard mRNA vaccines (6). Lower required doses could reduce pressure on manufacturing requirements and production needs when rolling out vaccines.
Additionally, one notable concern with mRNA-based COVID-19 vaccines has been their side effects including headaches, fatigue and fever. A lower dosage of an saRNA-based vaccine could mitigate those side effects. Further testing will be required to see if that happens in the clinic. In the published ARCT-154 trial results, there didn’t appear to be any clear difference in side effects compared to a standard mRNA COVID-19 vaccine (2).
Potential Drawbacks
saRNA includes genes that code for antigenic proteins and genes that encode replicase machinery. Consequently, this means saRNA vaccines contain much longer mRNA sequences than standard mRNA vaccines. The long length of saRNA makes it more challenging to manufacture and deliver (5). Producing long RNA constructs with high yields and purity is difficult (7) as is transporting a large, charged macromolecule across a cell membrane. Beisssert et al. suggested one solution is to produce trans-amplifying saRNA vaccines, which consists of two separate, shorter RNA fragments—a fragment encoding the antigen and a fragment encoding replicase (7).
There are also safety concerns with saRNA vaccines that will need to be monitored in the clinic. In immunocompromised individuals, for example, saRNA vaccines could persist longer because of less efficient clearance by their immune system. Similarly, safety trials in pregnant people will be needed to ensure that saRNA vaccines pose no additional risk to the fetus (5).
Another drawback that researchers will need to consider is that the intermediates produced during replication of saRNA can lead to an unfavorable immune response that prevents translation and successful immunization (1) or that leads to worse side effects (3). To avoid these responses, researchers will need to evaluate dose, delivery method and design of the saRNA (3).
Conclusion
The approval of an saRNA vaccine marks another milestone in the rapidly developing mRNA vaccine story. With several decades of research and the success of mRNA vaccines laying a solid foundation, we’re eager to see new research that expands on this technology, including replicon sequence optimization and the exploration of new applications in indications like cancers (5).
Interested in manufacturing mRNA? We offer cGMP-quality raw materials for cell-free mRNA therapeutic manufacturing that help you bridge the gap between discovery and commercialization.
References
- Bloom, K., van den Berg, F., Arbuthnot, P. (2021) Self-amplifying RNA vaccines for infectious diseases. Gene Ther. 28, 117. doi: 10.1038/s41434-020-00204-y
- Oda, Y. et al. Immunogenicity and safety of a booster dose of a self-amplifying RNA COVID-19 vaccine (ARCT-154) versus BNT162b2 mRNA COVID-19 vaccine: a double-blind, multicentre, randomized, controlled, phase 3, non-inferiority trial. (2023) Lancet Infect. Dis., in press. doi: 10.1016/S1473-3099(23)00650-3
- Tamás Szabó, G., Mahiny, A, J. and Vlatkovic, I. (2022) COVID-19 mRNA vaccines: platforms and current developments. Mol. Ther. 30, 1850. doi: 10.1016/j.ymthe.2022.02.016
- Hikke, M.C. and Pijlman, G.P. (2017) Veterinary Replicon Vaccines. Ann. Rev. Anim. Biosci. 5, 89. doi: 10.1146/annurev-animal-031716-032328
- Comes, J.D.G., Piglman, G.P. and Hick, T.A.H. (2023) Rise of the RNA machines–self-amplification in mRNA vaccine design. Trends in Biotech. 41, 1417. doi: 10.1016/j.tibtech.2023.05.007
- Vogel, A.B., et al. (2017) Self-amplifying RNA vaccines give equivalent protection against influenza to mRNA vaccines but at much lower doses. Mol. Ther. 26, 446. doi: 10.1016/j.ymthe.2017.11.017.
- Beissert, T., et al. (2020) A trans-amplifying RNA vaccine strategy for induction of potent protective immunity. Mol. Ther. 8, 119. doi: 10.1016/j.ymthe.2019.09.009
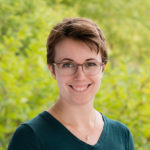
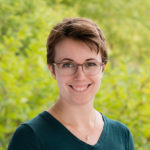
Latest posts by Jordan Nutting (see all)
- The Central Dogma of Promega: The Story and Science Behind Our Kit Packaging Design - May 7, 2024
- Silencing the Immunogenicity of AAV Vectors - April 4, 2024
- Discovering Cyclic Peptides with a “One-Pot” Synthesis and Screening Method - February 29, 2024