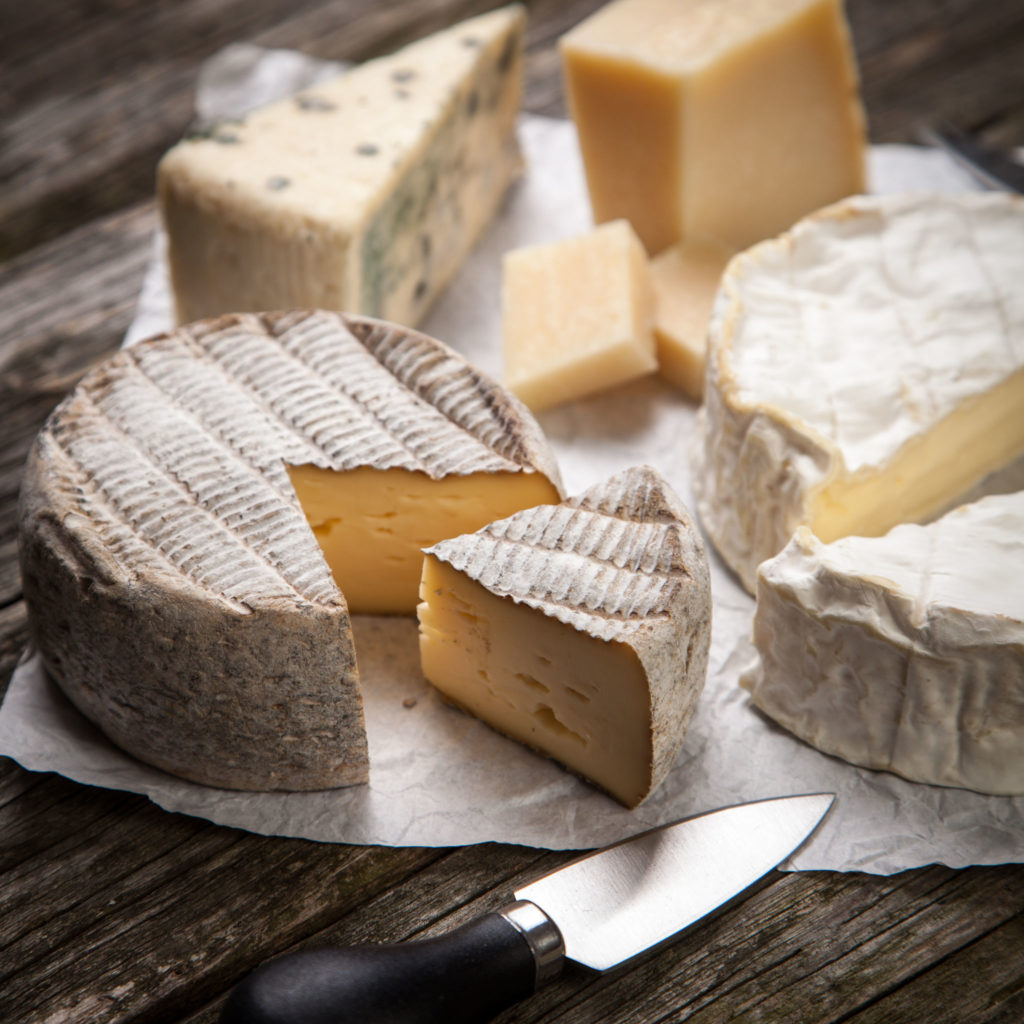
In almost every environment on earth, such as soil, human skin and gut, there lives a whole community of microbes—sometimes up to hundreds of species. It may seem like they all flourish in peace. But just like you may have friendly or hostile interactions with your neighbors, the different bacterial species interact in various ways. They may cooperate, compete or, sometimes, even kill each other. The interaction is complicated, and scientists have struggled to understand the nature of these microbiome interactions. How do microbiomes assemble and maintain stability? How do the interactions among different species affect gene expression?
To answer these questions, scientists first need a way to replicate a particular microbiome in the lab. To do this, they must be able to identify and grow every species within the microbiome in a controlled environment. Replicating most natural microbiomes is extremely difficult. But there’s one microbiome that’s easily replicated, simple to culture—and delicious—cheese!
The surface of cheese, known as the cheese rind, contains microbiomes that contribute to the unique smell and taste of the cheese. (Why does Limburger cheese smell like feet? Because the same bacteria on the cheese also lives on human skin and contribute to smelly feet.) Using cheese as a model system, researchers at the University of California, San Diego, investigated the genes that are upregulated in a multi-microbial environment.
The researchers first tweaked and tagged various E. coli genes to create mutants. The mutant bacteria were grown on cheese curd agar (yes, it’s a thing) either alone, paired with one other species, or in a community of three species. Using a new method called random barcode transposon-site sequencing (RB-TnSeq), they uncovered which genes that were most important for survival in the cheese rind microbiome.
The researchers found that the gene expression profile of paired microbes often changed when additional species were added. Some genes were only active when grown in a larger community. Overall, half of the microbiome interactions were attributed to interactions between two species, and the other half were more complex interactions.
The study also revealed different mechanisms of interactions, such as cooperation and competition. One example of cooperation is E. coli grown with fungi. When E. coli was grown alone, genes required for amino acid biosynthesis were highly expressed because amino acids are crucial for survival. However, when E. coli was grown with fungi, the amino acid synthesis pathway was downregulated. This suggests that fungi helped increase free amino acids within the environment so there is less need for E. coli to produce amino acids on their own. Results also showed that various species compete for iron, which is an essential nutrient for microbial growth but limited in the environment.
What’s exciting about this study is that it is the first to pinpoint the actual genetic interactions within a complex, albeit simplified, microbial community. It’s the first step in understanding complex microbial interactions and how they impact the survival of microbial communities. The researchers hope to use this new method to understand other microbiomes. The insights may even apply to clinical research, such as bacterial infections in human patients. So many possibilities—and it all started with cheese!
Related Posts
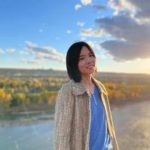
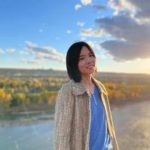
Latest posts by Johanna Lee (see all)
- Bacteria From Insect Guts Could Help Degrade Plastic - January 28, 2025
- A Diabetes Drug, Metformin, Slows Aging in Male Monkeys - December 19, 2024
- Understanding Persistent Digestive Disorders With Multiplex PCR - August 22, 2024