“The Andromeda Strain”, a novel written by Michael Crichton, remains one of my favorite science fiction novels for two reasons (spoiler alert for the plot): The US government deliberately sent objects into space to scoop up extraterrestrial microorganisms and examine their potential to be used as a weapon (with the expected consequences of contaminated space probes falling near human habitats and causing trouble), and the deadly organism infecting humans is stopped in its tracks by the inescapable bounds of its pH requirements exemplified by two survivors in an afflicted town: a crying baby and a Sterno-drinking man. Reality may be a bit different from the novel but the principle is the same: We are launching probes from our planet and sending them to other planetary bodies, sometimes to stay on another planet, sometimes to return to Earth. In both cases, worries about terrestrial organisms contaminating other planets and extraterrestrial organisms contaminating Earth are valid. Because we are sending more and more probes to examine the possibility of life on other planetary bodies, Curiosity being the most recent example, the question remains: How do you adequately test for organisms that may be hitching a ride from Earth into space?
All space faring agencies including National Aeronautics and Space Administration (NASA) have planetary protection policies in place that test for biological contamination of spacecraft surfaces during assembly, testing and launch operations. However, recent research suggested the methods used to sample microorganisms on clean room surfaces does not adequately represent the microbial diversity that may be present. To better test for the complete spectrum of organisms, the National Research Council has recommended archiving the organisms present on spacecraft and clean room surfaces as well as using molecular methods to improve phylogenic assessment of the microbes. Looking to improve testing methodology and analysis of possible biological contamination of space probes, Kwan et al. examined sampling methods, solvents and cryostorage for collecting, processing and analyzing microorganisms that may be present on clean room surfaces and contaminating launching spacecraft.
First the researchers created an 11 strain model microbial community (MMC) to test on the surfaces. These organisms included bacteria, archaea, fungi, aerobes, anaerobes, spores and nonspore-forming vegetative cells that have been found on space craft or in assembly clean rooms. Previous testing used only spores or cultured cells as positive controls and were not truly representative of the variety of microbal populations typically found in such environments. The MMC used for testing was prepared as a mixture of 1 × 106 cells and 1 × 105 spores per milliliter (11 strains × 105 cells/spores per ml each) in PBS, mixed well for an hour, and aseptically dispensed as 1.2ml aliquots of MMC into cryogenic vials. These mixtures were either immediately analyzed or stored at −80°C.
The surface used as the stand in for a space probe were two sizes of stainless steel grade 304-020 (304 brushed stainless steel sheet, 20 gauge, 0.036 inches thick): 25cm2 coupons and 2,500cm2 sheets. These surfaces were ultracleaned and tested to ensure no genetic material or organisms remained before storing in sterile containers until needed. MMC (1ml) in water or PBS was deposited onto either coupons (20 × 50μl drops) or sheets (50 × 20μl drops) using a multichannel electronic pipette. Then the stainless steel surfaces were covered and allowed to dry for 24 hours. Having the MMC dry on the surface of the stainless steel more closely mimics the desiccation to which the organisms would be subjected in reality.
Sampling of surfaces was methodical. For the 25cm2 coupons, either cotton or nylon-flocked swabs were moistened with PBS and moved first horizontally, then vertically and finally diagonally across the surface, rotating the swab with each pass. The swab head was then snipped and dropped into 10ml PBS for extraction of the microorganisms. For the larger 2,500cm2 sheets, either polyester wipes or BiSKits, a sponge collection unit, were wiped across the surface using the same directional swiping methodology as the swabs. PBS was used to remove the sampled microbes from both sampling materials, and the volumes for all swab, wipe and sponge samples were filter concentrated from milliliter volumes to microliters. Each final volume from the filter was diluted to 1.5ml, and one-third volume (0.5ml) was beaten with glass beads before a second untreated 0.5ml was mixed with the bead beaten volume, and DNA was extracted from the 1ml sample using Maxwell® 16 Cell DNA Purification Kit on the Maxwell® 16 Instrument. Automation was used for this step to maximize DNA recovery and minimize variability.
To assess the cryogenically stored samples, the total cell or spore numbers were counted under light microscopy over 90 days with the total counts remaining the same. rRNA qPCR analysis of purified DNA from the archived samples revealed a slight increase in yield for the first eight days of storage then remained steady up to the 90-day testing period. The yield increase is not surprising as a freeze-thaw cycle can enhance rupture of cells thus increasing nucleic acid accessibility to DNA isolation methods.
One interesting observation was that the MMC dispensed in PBS formed crystals on the surface of the stainless steel and inhibited microbe recovery during sampling. In fact, the MMC in water that was dispensed onto the test surfaces was recovered at 150 times greater efficiency compared to MMC dispensed in PBS. The researchers also tested if using sonication would enhance recovery of the MMC and found no effect on the ability to culture the MMC organisms. Nor were there any alterations in DNA integrity from samples subjected to sonication. In fact, the recovery of MMC as analyzed by DNA purification after sampling, filtration and concentration was approximately 60%, an level not reached by other methods used.
For the 25cm2 surface samples, cotton swabs recovered 32.8% of MMC rRNA abundance versus the significantly lower 4.3% recovery for nylon-flocked swabs as determined by qPCR. However, the signal-to-noise ratio (noise being contaminating DNA) found with cotton swab sample negative controls was high (19.6); nylon-flocked swab negative controls had a much lower ratio (0.6). Of note was a wide range of values from both cotton (104 to 105 rrn copies/25cm2) and nylon-flocked (103 to 105 rrn copies/25cm2) swab sampling replicates, but the authors could only suggest reasons why (e.g., variability in the swab or stainless steel surface).
The same analysis was performed for the 2,500cm2 surface samples. MMC rRNA abundance was determined using qPCR with BiSKit sponge recovery assessed at 41.9% compared to 26.7% recovery for polyester wipes. The signal-to-noise ratio for the polyester wipe negative controls (4.7) was high compared to the BiSKit negative controls ratio (1.1). The BiSKit sampling replicates had a range of 104 to 105 rrn copies/2,500cm2 but again there were only suggestions to explain this variability.
Then Kwan et al. assessed the recovery of each of the 11 organisms that made up the MMC using species-specific primers for rRNA and performing PCR once the species-specific PCR (ssPCR) detection limits for each individual organism were determined. Each sampling replicate was amplified and the PCR products analyzed on an Agilent 2100 Bioanalyzer. The ssPCR results reflected that of the rRNA qPCR. That is, cotton swabs recovered more MMC DNA (66.2%) compared to nylon-flocked swabs (48.1%), and BiSKits (55.8% recovery) performed better than polyester wipes (40.3% recovery) in MMC ssPCR. Only one organism was recovered in all sampling replicates: Bacillus megaterium KL-197. Not unexpectedly, two desiccation tolerant organisms, Deinococcus radiodurans R1 and Acinetobacter radioresistens 50v1, were amplified from a majority of the swab and BiSKit replicates. Two organisms were never recovered in any samples: Aureobasidium pullulans 28v1 and Staphylococcus warneri 82-4. However, these latter two organisms also had high limits of detection (9.0 × 106 and 4.3 × 104, respectively). Interestingly, Clostridium sporosphaeroides could only be found in the small surface samples; no amplifiable DNA was found in the the large surface samples.
The authors of the Applied and Environmental Microbiology paper examined how well organisms could be recovered from surfaces that had been assembled in a clean room for launching into space. They showed that using an 11 strain model microbial community demonstrated vulnerabilities in sampling technique and recovery even using PCR analysis. No single methodology is foolproof but even with two surface sizes, two sampling methods per surface and seven replicates per sampling method, there was a great degree of variability and even some organisms that were undetectable using ssPCR. Certainly, refinement in the techniques can help but space agencies do need to know exactly what we are sending to Mars and other planetary bodies on our space probes to ensure we are not contaminating those precious samples that will help scientists determine what life if any existed in our solar system, or at least separate out which might have come from Earth and which resided on the planetary body.
Reference
Kwan K, Cooper M, La Duc MT, Vaishampayan P, Stam C, Benardini JN, Scalzi G, Moissl-Eichinger C, & Venkateswaran K (2011). Evaluation of procedures for the collection, processing, and analysis of biomolecules from low-biomass surfaces. Applied and environmental microbiology, 77 (9), 2943-53 PMID: 21398492
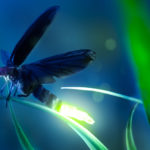
Sara Klink
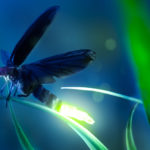
Latest posts by Sara Klink (see all)
- A One-Two Punch to Knock Out HIV - September 28, 2021
- Toxicity Studies in Organoid Models: Developing an Alternative to Animal Testing - June 10, 2021
- Herd Immunity: What the Flock Are You Talking About? - May 10, 2021
Acinetobacter? Deinococcus radiodurans? How fun to read a blog with those as subjects, Sara–thanks. Thought about this as well recently, with that bigger, better(?) Rover headed to Mars; what unexpected payload could be carried along? On the other hand one also wonders how easy it would be to keep lil’ ol’ Acinetobacter sp. alive for that two year(?) trip. Let’s hope not very (easy). kari