The global war against the coronavirus that causes COVID-19 rages on, spearheaded by efforts to develop effective and safe vaccines. At the time of writing, over 100 COVID-19 vaccine clinical trials were listed in the clinicaltrials.gov database. Recent attention has focused on mRNA vaccines developed by Pfizer/BioNTech and Moderna. If licensed, they would become the first mRNA vaccines for human use.
Other vaccine development efforts are relying on more conventional techniques—using an adenoviral vector to deliver a DNA molecule that encodes the SARS-CoV-2 spike (S) protein. Examples of these adenoviral vector vaccines include the vaccines from Oxford University/AstraZeneca (the UK), Cansino Biologics (China), Sputnik V (Russia) and Janssen Pharmaceuticals/Johnson & Johnson (the Netherlands and USA).
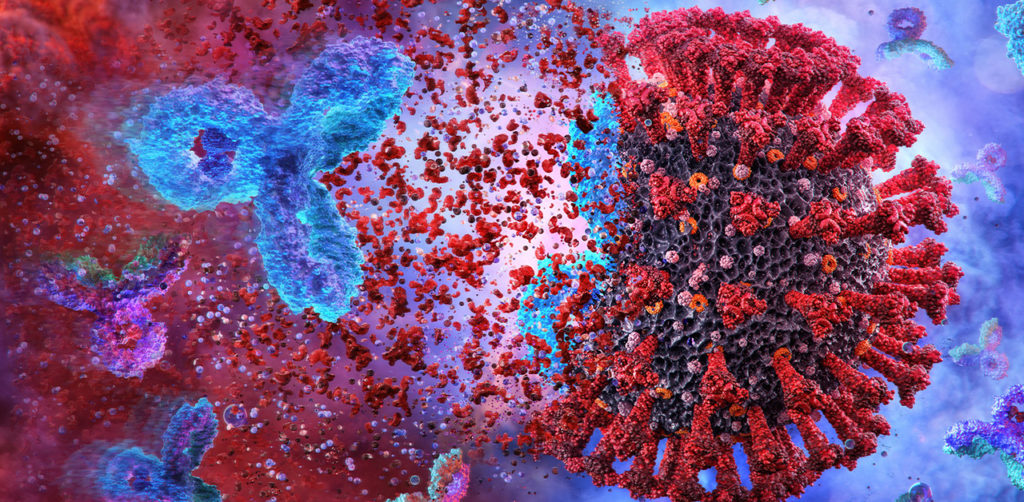
What Are Adenoviruses?
The first adenovirus was isolated in 1953 from human adenoid tissue; hence the name (1). Human adenoviruses belong to seven known species within the Mastadenovirus genus, and over 60 subtypes have been classified. They are associated with a variety of diseases that affect the respiratory or gastrointestinal systems, mostly in young children (reviewed in 2). Adenoviruses have a distinctive icosahedral structure, with a protein coat that encapsulates the viral double-stranded DNA genome. The adenovirus genome is relatively compact, making it an attractive option for insertion of foreign DNA. Deletion of the adenovirus E1A gene removes the virus’ ability to replicate. This ability can be restored during propagation in cell culture, for example, by using cells that express the E1A protein (3).
Early studies attempted to use adenoviral vectors for gene therapy—trying to compensate for a genetic defect by delivering copies of the correct DNA sequence into cells. These methods were largely unsuccessful, but the problem in this scenario became a solution in another. The failure of the first gene therapy experiments was attributed to the strong immune response raised by the adenoviral vectors that were used for gene delivery (3). However, a strong immune response is precisely what’s needed when developing a vaccine—and so, adenoviral vector vaccines were born.
How Do Adenoviral Vector Vaccines Work?
The strong adaptive immune response to adenoviruses is directed primarily against several antigens in the protein coat. This response includes both humoral (or antibody-mediated) and cellular immunity.
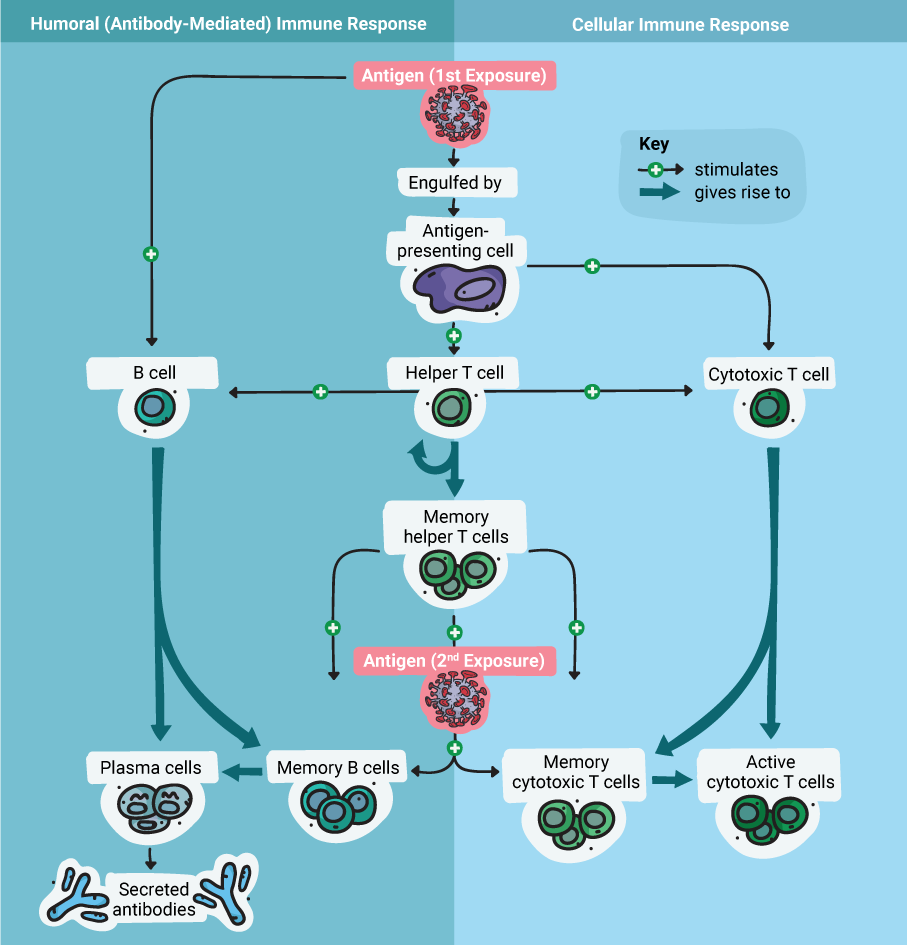
The humoral immune response ultimately results in the production of antibodies against the antigen(s), while the cellular response results in the production of specialized T cells. During future exposure to the antigen, the antibodies will bind to it, and the T cells will recognize and destroy any cells infected with a virus that carries the antigen. These actions combine to neutralize a virus quickly, before the infection spirals out of control.
Therefore, designing an adenoviral vector vaccine is (in theory) as simple as engineering the viral genome to produce a foreign antigen—for example, the coronavirus S protein. Once that’s done, the engineered adenovirus can be delivered as a vaccine through injection. Because the virus is replication-deficient, there’s no danger of it causing an infection. However, it can express the coronavirus S protein within the host cell, which then triggers the adaptive immune response to protect against an actual coronavirus infection. Essentially, this is the principle on which all vaccines are based: fooling the immune system into thinking there’s an infection, so that it can respond and be ready when a real infection occurs in the future.
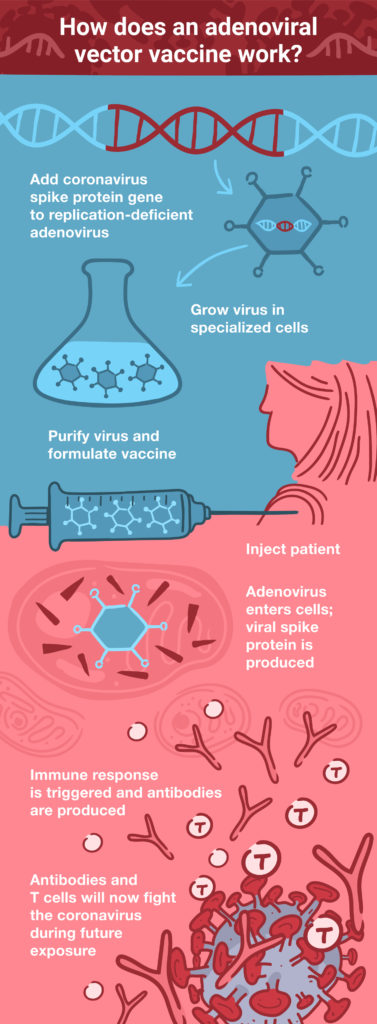
Despite their significant advantages—including stability, ease of large-scale production, and ability to accommodate large pieces of foreign DNA, adenoviral vector vaccines do have their problems. Initial concerns about the possibility of the viral DNA integrating into the host genome turned out to be largely unfounded (4). However, in rare cases, replication-competent adenoviruses can emerge through recombination during propagation in cell culture (5), but not in humans after injection.
Since human adenoviruses are so common, it’s possible that potential vaccine recipients could have pre-existing immunity, which would negate the effects of a vaccine (6). The best-characterized human adenovirus for vaccine development is species C, type 5 (hAd5); however, it’s also the most common (7). One solution to this problem is to use a less common subtype, such as hAd26, as was done to develop the Janssen vaccine against Ebola virus (8). The Sputnik V vaccine uses a combination of hAd5 and hAd26 (9). Another approach involves using an adenovirus from a different species; the Oxford/AstraZeneca COVID-19 vaccine is based on a chimpanzee adenovirus (10).
Designing an Effective Vaccine
Many considerations go into the design of recombinant adenoviral vectors to improve their efficacy and safety (reviewed in 11). Consideration should also be given to optimal design of the antigen. In the case of SARS-CoV-2, the coronavirus surface spike (S) protein is the antigen targeted by most vaccine development efforts. A recent collaborative study by groups that developed the Janssen COVID-19 vaccine provided insight into the antigen design process (12).
The S protein mediates SARS-CoV-2 entry into the cell after binding to the angiotensin-converting enzyme 2 (ACE2) receptor on the host cell surface. It is comprised of two subunits, S1 and S2. S1 binds to the ACE2 receptor, while cleavage of S2 by proteases on the host cell surface alters its conformation and enables the viral envelope to fuse with the cell membrane. Before this fusion event, the S protein exists in a so-called metastable prefusion conformation, and proteolytic cleavage refolds the protein into a more stable, postfusion state (13).
Bos et al. designed and expressed several variants of the S protein, along with the wild-type protein, to examine the stability and immunogenicity of each variant (12). They tested the binding properties of the expressed antigens by cell-based ELISA and flow cytometry using neutralizing ligands (ACE2 receptor binding domains) and antibodies to identify the candidate antigen with the greatest prefusion stability. Further mutational analysis aimed to optimize the N-terminal signal peptide that ensures correct cleavage and folding of the N-terminal domain. Cotransfection experiments with the S protein variants and ACE2, the cell-surface protease, and green fluorescent protein (GFP) provided further information on the optimal antigen variants. To increase the sensitivity of this assay, the researchers redesigned it using NanoBiT® complementation technology instead of GFP as the reporter. These results were supplemented by in vivo experiments in mice to assess live virus neutralization, humoral and cellular immunogenicity, and T-cell response to determine the risk of vaccine-associated enhanced disease.
The results identified S protein variants with stabilizing mutations that optimized prefusion stability and produced the best ratio of neutralizing to non-neutralizing antibodies. However, the wild-type signal peptide sequence proved to be optimal for correct protein folding. Based on their research, the group proceeded with the development of an optimal Ad26-based viral vector vaccine, which is currently being evaluated in a phase 1 clinical trial.
What’s Next?
COVID-19 vaccine development and clinical trials continue to move forward at an unprecedented pace. Both mRNA and adenoviral vector vaccines will be vital in combating this global pandemic. In the US, the Pfizer/BioNTech and Moderna mRNA vaccines have received US Food and Drug Administration (FDA) emergency authorization approval. AstraZeneca is considering a collaboration with the Sputnik V developers on a combination vaccine. Meanwhile, after an initial pause, the Janssen/J&J phase 3 trial of its original Ad26-based vaccine is set to resume.
With any virus, new, mutated strains can emerge globally, and such is the case with SARS-CoV-2. Recent evidence of mutation rates being more rapid than previously thought have raised concerns in the UK. Despite some of the mutations being mapped to the S protein, it is likely that current vaccines will still prove effective. As the virus evolves, so must vaccine design and development. Multiple approaches to vaccine development and distribution will provide the best hope of tackling this pandemic—and the next one.
Learn more about viral vaccine development with our SARS-CoV-2 Research, Vaccine and Therapeutic Development resource page.
References
- Rowe, W.P. et al. (1953) Isolation of a cytopathogenic agent from human adenoids undergoing spontaneous degeneration in tissue culture. Proc. Soc. Exp. Biol. Med. 84, 570–573.
- Ghebremedhin, B. (2014) Human adenovirus: viral pathogen with increasing importance. Eur. J. Microbiol. Immunol. 4(1), 26–33.
- Tatsis, N. and Ertl, H.C.J. (2004) Adenoviruses as vaccine vectors. Mol. Ther. 10(4), 616–629.
- Hillgenberg, M. et al. (2001) Chromosomal integration pattern of a helper-dependent minimal adenovirus vector with a selectable marker inserted into a 27.4-Kilobase genomic stuffer. J. Virol. 75, 9896–9908.
- Fallaux, F.J. et al. (1999) Who’s afraid of replication-competent adenoviruses? Gene Ther. 6, 709–712.
- Fausther-Bovendo H. and Kobinger, G.P. (2014) Pre-existing immunity against Ad vectors: humoral, cellular, and innate response, what’s important? Hum. Vaccin. Immunother. 10, 2875–2884.
- Mast, T.C. et al. (2010) International epidemiology of human pre-existing adenovirus (Ad) type-5, type-6, type-26 and type-36 neutralizing antibodies: Correlates of high Ad5 titers and implications for potential HIV vaccine trials. Vaccine 28, 950–957.
- Custers, J. et al. (2020) Vaccines based on replication incompetent Ad26 viral vectors: Standardized template with key considerations for a risk/benefit assessment. Vaccine (in press; JVAC 22294).
- Lagunov, D.Y. et al. (2020) Safety and immunogenicity of an rAd26 and rAd5 vector-based heterologous prime-boost COVID-19 vaccine in two formulations: two open, non-randomised phase 1/2 studies from Russia. Lancet 396, 887–897.
- Voysey, M. et al. (2020) Safety and efficacy of the ChAdOx1 nCoV-19 vaccine (AZD1222) against SARS-CoV-2: an interim analysis of four randomised controlled trials in Brazil, South Africa, and the UK. Lancet, published online December 8, 2020.
- Afkhami, S. (2016) Methods and clinical development of adenovirus-vectored vaccines against mucosal pathogens. Mol. Ther. Meth. Clin. Dev. 3, 16030.
- Bos, R. et al. (2020) Ad26 vector-based COVID-19 vaccine encoding a prefusion-stabilized SARS-CoV-2 Spike immunogen induces potent humoral and cellular immune responses. npj Vaccines 5, 91.
- Sternberg, A. and Naujokat, C. (2020) Structural features of coronavirus SARS-CoV-2 spike protein: Targets for vaccination. Life Sci. 257, 118056.
Related Posts
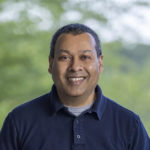
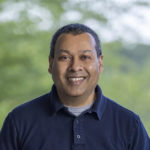
Latest posts by Ken Doyle (see all)
- Will Artificial Intelligence (AI) Transform the Future of Life Science Research? - February 1, 2024
- RAF Inhibitors: Quantifying Drug-Target Occupancy at Active RAS-RAF Complexes in Live Cells - September 5, 2023
- Synthetic Biology: Minimal Cell, Maximal Opportunity - July 25, 2023
The CDC website’s section on viral vector vaccines, says that the viral gene shuttled by the adenovirus does not enter the nucleus and does not get integrated into hostd DNA. How then do we get from viral genome to spike proteins? Can transcription of the viral gene occur outside the nucleus?
Thank you for your comments. Adenoviral DNA does not integrate into the host cell genome but remains in an episomal state within nondividing cells. It is transcribed by the host cell RNA polymerase complex within the nucleus, and the RNA is then translated in the cytoplasm as usual.
It can happen in the cytoplasm, I believe.
How long does the adenovirus DNA stay in the nucleus? And does it pass on to offsprings? Thanks!
The lifetime of episomal adenoviral DNA in the nucleus varies depending on the sequence, cell type, and other factors (reviewed by Lee et al: https://www.ncbi.nlm.nih.gov/pmc/articles/PMC5609467/). Episomal DNA is lost during cell division, so it is not passed on to daughter cells
What about non dividing cells like neurons and cardiomyocytes. Do we know if the transgene expression is transient or continuous?
I think there are cases where episomal DNA can be persistent, like the cccDNA of Hepatitis-B retroviruses (causing chronic carriers). How are adenoviruses different? Are they really harmless in this respect? Thank you.
“Please see reference 11 in the post and also this review by Lee et al. https://www.ncbi.nlm.nih.gov/pmc/articles/PMC5609467/“
Oh My God!!! This is a great blog, I am happy that I have come across this one. It’s an amazing blog to read, so many things about covid-19 vaccines adenoviral vectors in India. Thanks for this wonderful content. Do check out this https://peerlessengineering.com/ it has some great and nice ideas to look for.
With the large difference in the efficacy (and possibly even a significant safety concern with blood clotting emerging and pending investigations from the Oxford adenovirus vaccine) rates between the mRNA and virus-vectored DNA vaccines, I cannot see how vaccine-makers can quixotically and stubbornly insist on developing and producing these DNA vaccines, notwithstanding its easier and cheaper scaling and mass production advantages, which I only attribute to the initial lack of infrastructure and experience that come naturally with new technology platforms that should go away eventually. I also do not quite trust that the emergence of replication-competent adenovirus that happens in vitro via recombination cannot then possibly happen in vivo despite the initial lack of evidence in a few examples after introduction to hundreds of millions if not billions of people, especially with how ubiquitous adenoviruses are in humans. Same goes – due to the possible recombination – for the viral DNA to be presumed to remain permanently stored harmlessly in its episomal enclave in the nucleus. A corollary to the combination of Occam’s Razor and Adam Smith’s “Invisible Hand” working synergistically should give the final blow to any remaining romantic delusions in pursuing something that has repeatedly failed spectacularly or looked foolish in comparison.
My question then is what could possibly be the reasons as to why the “mRNA enclosed in lipid-nanoparticle-vehicle technology” has so far emerged the clear victor by heads and shoulders above its competitors despite the theoretical straightforwardness and mimicry of natural processes of fhe viral vector approach? Why would the introduction of the very protein antigens themselves (e.g. Sinovac), that has been the essence of vaccination from when it was first thought of centuries ago, be far less efficacious than introducing the final genomic code for them into our bodies?
Question regarding adenoviral integration. In the article, you state that initial concerns were ‘largely unfounded’ and cite reference (4). However, that paper by Hillgenberg et al confirms that viral vector integration DOES in fact occur, albeit in an HDR-independent fashion. I think it’s particularly concerning that they’re observing integration with even E1-deleted AdV. To quote their final paragraph:
“(i) as adenovirus vectors, they infect a large variety of cell types at high efficiency; (ii) they obviously integrate at high efficiency, primarily as one complete monomer per cell; (iii) the high capacity of these vectors could enable stable transfer of genes together with complex regulatory elements to achieve regulated or tissue specific expression; and (iv) adverse effects mediated by residual adenovirus gene expression are excluded
Are there other references that you can share that definitively refute these findings?