Restriction enzymes sometimes get a lot of flak. In the not-so-distant past, they were the workhorses of molecular biology. Restriction enzymes played a huge role in developing early DNA sequencing techniques. They chop DNA in a predictable manner, which makes cutting and pasting genes of interest manageable and relatively easy, enabling the development of genetic engineering and recombination technologies. These technologies are now moving beyond restriction enzymes toward more modern methods, with the most talked-about method being CRISPR /Cas9. As technology continues to advance at such a rapid pace, restriction analysis and other “ancient” technologies feel antiquated. But this is not necessarily the case.
Continue reading “Think Restriction Enzymes are so last decade? Not so fast!”Tips and Tools
Restriction Enzyme Digestion: Capabilities and Resources
Restriction enzymes recognize short DNA sequences and cleave double-stranded DNA at specific sites within or adjacent to these sequences. These enzymes are the workhorse in many molecular biology applications such as cloning, RFLP, methylation-specific restriction enzyme analysis of DNA, etc. Restriction enzymes with enhanced capabilities can help you streamline and shorten these workflows and improve success of restriction enzyme digestion.
A subset of Promega restriction enzymes offer capabilities that include rapid digestion of DNA in 15 minutes or less, ability to completely digest DNA directly in the GoTaq® Green Master Mix, and Blue/White Cloning Qualification which allows for rapid, reliable detection of transformants.
To learn more about restriction enzymes and applications, check out Restriction Enzyme Resource on the web. The resource provides everything from information on restriction enzyme biology to practical information on how to set up and design a restriction enzyme digestion. This resource also contains useful online tools, including the Restriction Enzyme Tool, to help you use enzymes more effectively. It helps you choose the best reaction buffer for double digests, find the commercially available enzyme that cuts your sequence of interest, find compatible ends, and search for specific information on cut site, overhang isoschizomers and neoschizomers by enzyme name.
For added convenience, you can download the mobile app available for iOS devices and use the Restriction Enzyme Tool to plan your next digest.
For additional information regarding Restriction Enzyme Digest, reference the supplementary video below.
Related Posts
A Quick Method for A Tailing PCR Products
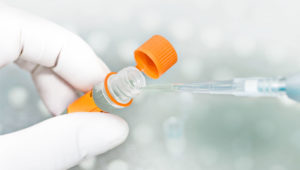
Some thermostable DNA polymerases, including Taq, add a single nucleotide base extension to the 3′ end of amplified DNA fragments. These polymerases usually add an adenine, leaving an “A” overhang. There are several approaches to overcome the cloning difficulties presented by the presence of A overhangs on PCR products. One method involves treating the product with Klenow to create a blunt-ended fragment for subcloning. Another choice is to add restriction sites to the ends of your PCR fragments. You can do this by incorporating the desired restriction sites into the PCR primers. After amplification, the PCR product is digested and subcloned into the cloning vector. Take care when using this method, as not all restriction enzymes efficiently cleave at the ends of DNA fragments, and you may not be able to use every restriction enzyme you desire. There is some useful information about cutting with restriction sites close to the end of linear fragments in the Restriction Enzyme Resource Guide. Also, some restriction enzymes require extra bases outside the recognition site, adding further expense to the PCR primers as well as risk of priming to unrelated sequences in the genome.
Continue reading “A Quick Method for A Tailing PCR Products”Selecting the Right Colony: The Answer is There in Blue and White
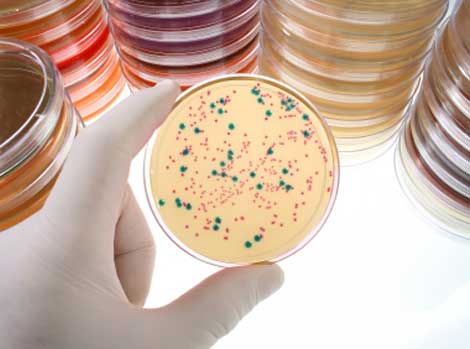
Ah, the wonders and frustrations of cloning. We’ve all been there. After careful planning, you have created the cloned plasmid containing your DNA sequence of interest, transformed it into bacterial cells and carefully spread those cells on a plate to grow. Now you stand at your bench gazing down at your master piece: a plate full of tiny bacterial colonies. Somewhere inside those cells is your DNA sequence, happily replicating with its plasmid host. But wait – logic tells you that not ALL of those colonies can contain your plasmid. There must be hundreds of colonies. Which ones have your plasmid? You begin to panic. Visions of yourself old and grey and still screening colonies flash through your mind. At the next bench, your lab-mate is cheerfully selecting colonies to screen. Although there are hundreds of colonies on her plate as well, some are white and some are blue. She is only picking the white colonies. What does she know that you don’t?
Continue reading “Selecting the Right Colony: The Answer is There in Blue and White”Lab Sustainability: Easy as 1-2-3
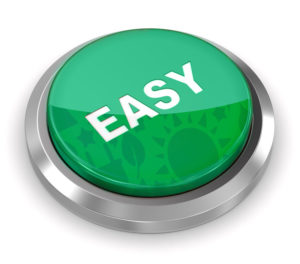
Sustainability is a bit of buzzword lately—for good reason—but knowing how to be more sustainable and actually putting sustainable practices in action are not the same thing. This may be one reason why scientists have been slow to adopt change in their laboratories. By sponsoring My Green Lab, we’re hoping to help spread the message that there are simple changes researchers can make in their labs to significantly impact sustainability.
Here are some easy ways to reduce energy, water and waste in your lab and start making your research more sustainable.
1. Energy
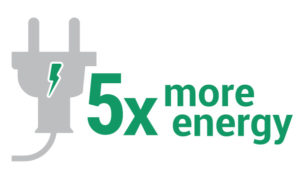
Compared to office buildings on campus, academic lab buildings consume 5 times more energy. To put that into perspective, labs typically consume 50% of the energy on a university campus despite occupying less than 30% of the space. Fortunately, reducing energy usage can be one of the easiest ways to make your lab more sustainable.
Continue reading “Lab Sustainability: Easy as 1-2-3”Why You Don’t Need to Select a Wavelength for a Luciferase Assay
It’s a question I’m asked probably once a week. “What wavelength do I select on my luminometer when performing a luciferase assay?” The question is a good and not altogether unexpected one, especially for those new to bioluminescent assays. The answer is that in most cases, you don’t and in fact shouldn’t select a wavelength (the exception to this rule is if you’re measuring light emitted in two simultaneous luciferase reactions). To understand why requires a bit of an explanation of absorbance, fluorescence, and luminescence assays, and the differences among them.
Absorbance, fluorescence, and luminescence assays are all means to quantify something of interest, be that a genetic reporter, cell viability, cytotoxicity, apoptosis, or other markers. In principle, they are all similar. For example, a genetic reporter assay is an indicator of gene expression. The promoter of a gene of interest can be cloned upstream of a reporter such as β-galactosidase, GFP, or firefly luciferase. The amount of each of these reporters that is transcribed into mRNA and translated into protein by the cell is indicative of the endogenous expression of the gene of interest.
Continue reading “Why You Don’t Need to Select a Wavelength for a Luciferase Assay”What Makes a “Good” Buffer?
Buffers are often overlooked and taken for granted by laboratory scientists, until the day comes when a bizarre artifact is observed and its origin is traced to a bad buffer.
The simplest definition of a buffer is a solution that resists changes in hydrogen ion concentration as a result of internal and environmental factors. Buffers essentially maintain pH for a system. The effective buffering range of a buffer is a factor of its pKa, the dissociation constant of the weak acid in the buffering system. Many things, such as changes in temperature or concentration, can affect the pKa of a buffer.
In 1966, Norman Good and colleagues set out to define the best buffers for biochemical systems (1). By 1980, Good and his colleagues identified twenty buffers that set the standard for biological and biochemical research use (2,3). Good set forth several criteria for the selection of these buffers:
Continue reading “What Makes a “Good” Buffer?”Eight Considerations for Getting the Best Data from Your Luminescent Assays
The stage is set. You’ve spent days setting up this experiment. Your bench is spotless. All the materials you need to finally collect data are laid neatly before you. You fetch your cells from the incubator, add your detection reagents, and carefully slide the assay plate into the luminometer. It whirs and buzzes, and data begin to appear on the computer screen. But wait!
Don’t let this dramatic person be you. Here are 8 tips from us on things to watch out for before you start your next luminescent assay. Make sure you’ll be getting good data before wasting precious sample!
Continue reading “Eight Considerations for Getting the Best Data from Your Luminescent Assays”
Converting RPM to g Force (RCF) and Vice Versa
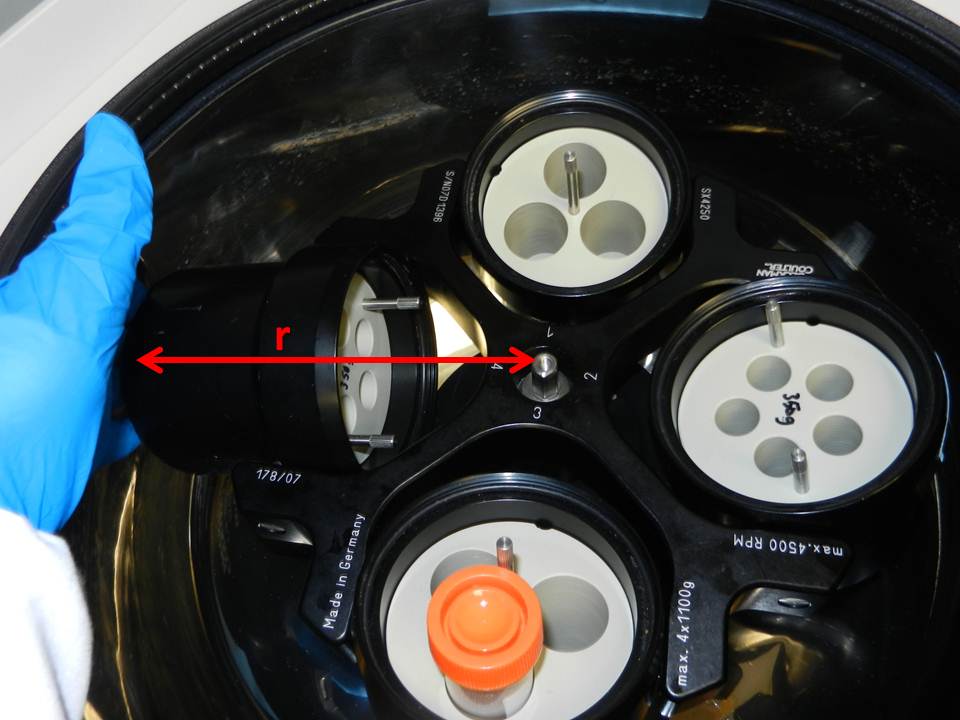
g Force or Relative Centrifugal Force (RCF) is the amount of acceleration to be applied to the sample. It depends on the revolutions per minute (RPM) and radius of the rotor, and is relative to the force of Earth’s gravity.
A good, precise protocol for centrifugation instructs you to use the g force rather than RPMs because the rotor size might differ, and g force will be different while the revolutions per minute stay the same. Unfortunately, many protocols are written in hurry and instructions are given in RPMs. Therefore, you have to convert g force (RCF) into revolutions per minute (rpms) and vice versa.
Modern centrifuges have an automatic converter but older ones do not. There is a simple formula to calculate this, but it takes some time to do the calculation. Meanwhile, your cells might die or the biochemical reaction goes on for three times longer than it should.
There are several ways to make conversion:
Continue reading “Converting RPM to g Force (RCF) and Vice Versa”In Vitro Transcription: Common Causes of Reaction Failure
A widely used molecular biology technique, in vitro transcription uses bacteriophage DNA-dependent RNA polymerases to synthesize template-directed RNA molecules. Enzymes like bacteriophage SP6, T3 and T7 RNA polymerases are used to produce synthetic RNA transcripts, which can be used as hybridization probes, as templates for in vitro translation applications, or in structural studies (X-ray crystallography and NMR). Synthesized RNA transcripts are also used for studying cellular RNA functionality in processes such as splicing, RNA processing, intracellular transport, viral infectivity and translation.
Problems in the transcription reaction can result in complete failure (i.e., no transcript generated) or in transcripts that are the incorrect size (i.e., shorter or longer than expected). Below is a discussion of the most common causes of in vitro transcription problems.
Continue reading “In Vitro Transcription: Common Causes of Reaction Failure”