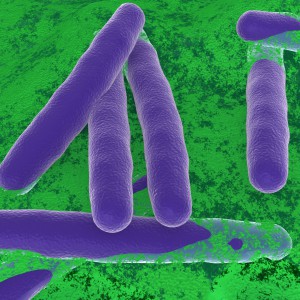
Microbial cells outnumber the cells of our own bodies approximately 10:1, these microbes that live on our skin and along the epithelial linings of our internal tubes make up our microbiota*, and they can have major effects on our health. Most of our microbiota are commensal organisms, living in harmony with our body, but if you suppress our immune system or greatly reduce their populations with large doses of antibiotics, and you will soon see the effects of disrupting our microbiota.
There is much interest in the microbiota that inhabit our bodies. For instance several studies have indicated that intestinal microbes can play a big part in obesity, with changes in the makeup of the microbiota being a major risk factor (1). But many of these organisms are hard to learn about—the ones that inhabit the deep folds of our gut thrive in moist, warm, anaerobic conditions with lots of specialized nutrients, conditions that are very hard to replicate in the laboratory. For that reason, we don’t know much about many of the microbes that are the most abundant within us.
The Human Microbiome Project begun in 2008 by the National Institutes of Health (2) seeks to understand human microbiota and their relationship to human health. To do this, the researchers leading the project took a metagenomic approach—using advanced DNA sequencing technologies to sequence the genomes of human microbiota and get a look at the human microbiome—without culturing the microbes.
But to truly understand their biology, and to perhaps exploit what we learn to enhance human health we need to be able to manipulate these organisms. In particular, biologists who are interested in synthetic biology would like to use these micro-organisms to monitor what is going on in our bodies, particularly our guts. What better monitor for these hard-to-access places than an organism that is already well adapted to live there?
Continue reading “Manipulating Microbiota: A Synthetic Biology Exploration of the Gut”