Cyanobacteria, microscopic photosynthetic bacteria, have been quietly shaping our planet for billions of years. Responsible for producing the oxygen we breathe, these tiny organisms play a critical role in the global carbon cycle and are now stepping into the spotlight for another reason: their potential to both understand and potentially combat climate change.
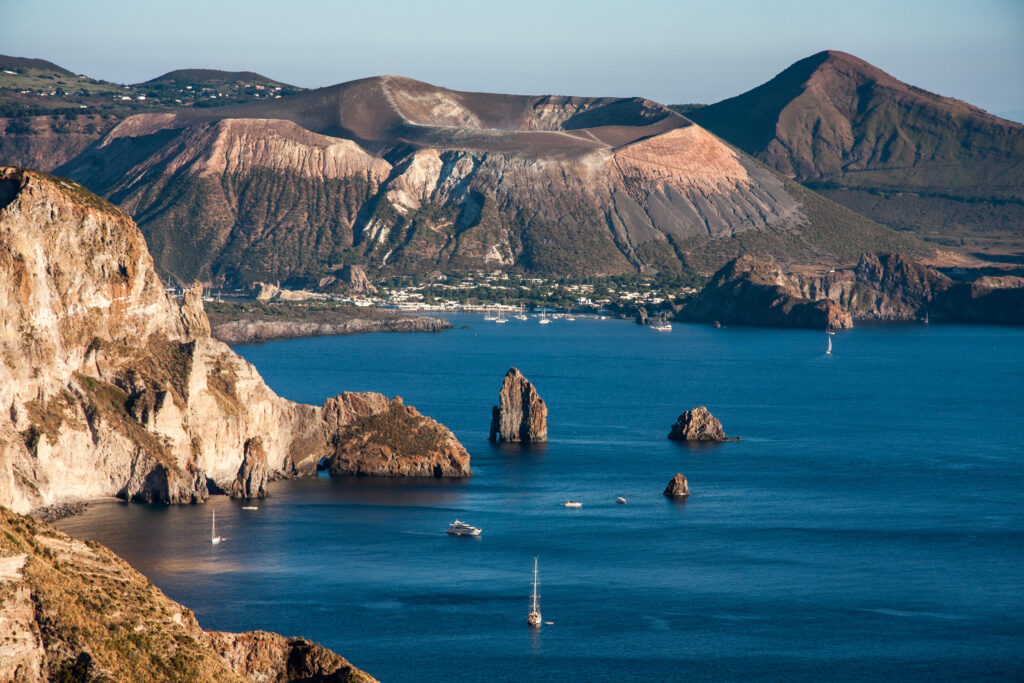
Recently, researchers discovered two new strains of cyanobacteria, UTEX 3221 and UTEX 3222, thriving in a marine volcanic seep off the coast of Italy. While cyanobacteria are virtually everywhere there is water and light—from calm freshwater ponds to extreme environments like Yellowstone’s hot springs—this particular habitat is remarkable for its naturally high CO₂ levels and acidic conditions. For these newly identified strains, a geochemical setting like marine volcanic seeps have likely driven the evolution of unique traits that could make them valuable for carbon sequestration and industrial applications.
How can something so small make such a big impact? In this blog, we explore what makes these newly discovered cyanobacteria special and how this research could help address some of the world’s most pressing challenges.
A Dense Discovery from the Depths of Baia di Levante
The Baia di Levante, nestled near Italy’s Vulcano Island, is a place where geology and biology collide. This shallow marine region is dotted with volcanic seeps that continuously release CO₂ into the water, creating an environment rich in CO₂ while maintaining an acidic (low) pH. Unlike deeper oceanic vents, where sunlight cannot penetrate, Baia di Levante’s shallow waters provide the key ingredient for photosynthesis: light. For most organisms, these conditions would pose significant challenges. But for photosynthetic microorganisms, these particular volcanic seeps offer an abundance of resources: CO₂, sunlight, and water.
In the hunt for novel photosynthetic species, researchers sampled from seeps in Baia di Levante and identified two novel cyanobacteria strains, UTEX 3221 and UTEX 3222. Among these, UTEX 3222 quickly emerged as a standout, showcasing a combination of traits that make it uniquely promising for research and industrial applications.
For starters, UTEX 3222 boasts a doubling time of just 2.35 hours—making it one of the fastest-growing cyanobacteria studied to date. In laboratory cultures, it produces over 31 grams of dry biomass per liter, nearly double the yield of some of the current model strains used in biotechnology. It thrives under diverse conditions, tolerating high salinity, varying pH levels, and elevated temperatures, all while maintaining robust growth.
There are a handful of common lab strains that researchers use to study cyanobacterial photosynthesis in situ. In comparison, UTEX 3222 is much larger than the common laboratory strain, Synechococcus elongatus. Furthermore, UTEX 3222’s cells are noticeably larger and form denser colonies. The strain also contains significantly more carbon, stored in visible white granules within its cells. Finally, researchers found that the strain was considerably heavier’ than S. elongatus: when placed in a test tube, UTEX 3222 rapidly sunk to the bottom, a deviation from other strains. Thus, UTEX 3222 was affectionately given the name “Chonkus” for its particularly dense phenotype.
Balancing Carbon Capture and Ecosystem Impact
Cyanobacteria have long been recognized for their versatility in industrial applications as they readily convert sunlight and carbon dioxide into biomass. Thus, these photosynthetic bacteria are often used as workhorses for synthesizing materials in a more sustainable way. Their use spans a wide range of industries, from producing biofuels and bioplastics to synthesizing valuable compounds like vitamins, pigments, and pharmaceuticals. In recent years, they’ve also gained attention for their potential in carbon capture technologies, where their rapid growth and high photosynthetic efficiency can play a pivotal role in reducing atmospheric CO₂ levels.
In industrial processes, biomass harvesting is often one of the most costly and resource-intensive steps. Traditional methods rely on chemical flocculants or complex filtration systems to separate cells from their growth medium. Chonkus, however, naturally settles to the bottom of a container within hours, forming a dense pellet. This trait could drastically reduce the time, energy, and cost required to collect biomass, making it an attractive candidate for large-scale bioproduction.
Beyond industry, Chonkus has profound implications for carbon sequestration. In natural ecosystems, cyanobacteria and other photosynthetic organisms play a critical role in the carbon cycle, capturing CO₂ from the atmosphere and converting it into biomass. However, much of this carbon is recycled back into the environment when these organisms die and decompose near an ocean or lake surface. The strain’s rapid settling behavior could change this dynamic. By sinking to deeper ocean layers, its biomass has the potential to transport carbon to regions where it can remain stored for centuries.
But carbon that sinks doesn’t simply vanish—it interacts with the ocean floor, an environment actively cycling other elements like nitrogen, phosphorus, and sulfur. Introducing large amounts of cyanobacterial biomass to these deep ecosystems could shift nutrient balances and alter microbial communities. While this presents exciting possibilities for carbon sequestration, it also highlights the need for careful study of long-term impacts on ocean nutrient cycling, geochemistry, and biodiversity.
This behavior is closely tied to the ocean’s biological pump, a natural process that moves organic carbon from the surface to the deep sea. Enhancing this process with organisms like Chonkus could offer a scalable tool for mitigating climate change by increasing the amount of carbon stored in deep ocean layers. However, as we explore these possibilities, it’s essential to understand how such interventions might affect nutrient cycling and biodiversity on the ocean floor. Balancing the promise of carbon sequestration with its ecological implications will be critical for leveraging photosynthetic bacteria in future applications.
Read more about this research in Science.