Back when I was a graduate student (more than a few years ago), I remember hearing another student joke that if a member of his thesis committee asked him to explain an unexpected or unusual result, he was going to “blame” epigenetics. At that time, the study of epigenetic gene regulation was in its infancy, and scientists had much to learn about this mysterious regulatory process. Fast forward to today, and you’ll find that scientists know a lot more about basic epigenetic mechanisms, although there is still plenty to learn as scientists discover that the topic is much more complicated than initially thought, as is often the case in science. A recent EMBO Journal article is contributing to our knowledge by shedding light on the role of the TET family of DNA-modifying enzymes in epigenetics (1).
Briefly, epigenetics refers to heritable changes in gene expression that arise from changes in chromosomes without alteration of DNA sequence. Epigenetic mechanisms include modifications to DNA and histone components of nucleosomes as well as expression of noncoding RNAs. DNA modifications usually involve DNA methylation on the 5C position of cytosine residues to yield 5-methylcytosine. Histone modifications abound, but the predominant modifications include acetylation, methylation, phosphorylation, ubiquitination and sumoylation, with thousands of potential combinations of modifications within a single nucleosome. One epigenetic mark worth noting for the purposes of this discussion is the transcription-friendly trimethylation of the lysine at position 4 of histone H3 (H3K4me3). Together these epigenetic modifications can affect gene accessibility to regulatory proteins, transcription factors, RNA polymerase II and other components of the transcriptional machinery, ultimately altering transcription patterns, often in tissue- and cell-specific ways.
The Ten-Eleven Translocation (TET) proteins are involved in this regulatory process, but scientists don’t yet have a clear understanding of their roles and mechanisms. TET proteins catalyze the oxidation of 5-methylcytosine to form 5-hydroxymethylcytosine, an epigenetic mark that is not well understood. TET1 has been linked to epigenetic repression complexes (2,3) and is important in the regulation of pluripotency of embryonic stems cells and cellular differentiation (2–5). TET2 plays a role in hematopoiesis, and TET3 is important for epigenetic reprogramming in mouse germ cells (6).
In the recent EMBO Journal paper, Deplus and her collaborators, some of whom are Promega scientists, set out to determine how TET2 and TET3 proteins are involved in epigenetic regulation. To characterize proteins that interact with TET, the authors expressed full-length TET1, TET2 and TET3 as HaloTag® fusion proteins and performed protein pull-downs. They identified novel interactions between all three TET proteins and O-GlcNAc transferase (OGT), which catalyzes the addition of N-acetylglucosamine (GlcNAc) to numerous transcription factors, regulatory proteins and histones to activate or inhibit the target protein or recruit additional proteins. In this paper, they focused on TET2 and TET3, which showed the strongest interaction with OGT. They mapped TET2, TET3 and OGT binding throughout the genome by expressing these proteins as HaloTag® fusion proteins in HEK293T cells, crosslinking the proteins and DNA, then capturing the fusion proteins and associated DNA fragments and performing high-throughput sequencing to show that TET2/3 and OGT colocalize at active gene promoters and were tightly clustered near transcription start sites.
What is TET2/3-OGT doing at these promoters? The simple answer is that these proteins are promoting transcription; the complete answer is more complicated. The authors re-examined their initial protein pull-down data and noticed that host cell factor 1 (HCF1), a known component of the H3K4 methyltransferase complex known as SET1/COMPASS and a known target for GlyNAcylation, also interacted with TET2/3 and OGT. Additional protein pull-down experiments showed that TET2/3-OGT interacts with all components of the SET1/COMPASS complex. By reducing TET2 and TET3 expression levels through RNA interference, they discovered that reduced TET expression lead to decreased OGT activity (but not OGT expression levels), reduced HCF1 GlycNAcylation and, because HCF1 GlyNAcylation is required to maintain the integrity of the SET1/COMPASS complex, lower levels of H3K4me3. So, less TET2/3 protein means less HCF1 GlycNAcylation, fewer SET1/COMPASS complexes, less H3K4me3 and lower transcription activity.
To assess whether TET2/3-OGT activity affects the interaction of SET1/COMPASS with chromatin, the authors used bioluminescence resonance energy transfer (BRET). They created a fusion protein consisting of the H3K4 methyltransferase SETD1A and NanoLuc® luciferase as the energy donor and a fluorescently labeled histone H3.3-HaloTag® fusion protein as the energy acceptor. If the SETD1A-NanoLuc® protein and fluorescently labeled H3.3 protein are in close proximity, energy is transferred from SETD1A-NanoLuc® to H3.3 and is detected as light emitted by the fluorescent label. When cells expressing these fusion proteins were treated with an OGT inhibitor or RNA molecules to knockdown TET2/3 expression, the BRET signal decreased compared to the signal in untreated cells. Lower signal indicates less interaction between the SET1/COMPASS complex and the H3.3 protein component of chromatin. These BRET experiments confirmed that TET2/3-OGT activity is necessary for SET1/COMPASS complex function and showed that TET and OGT activities promote binding of SETD1A, a component of the SET1/COMPASS complex, to chromatin. This binding increases H3K4me3 levels. Thus, the authors’ data support a TET2/3-OGT-mediated mechanism for regulating the SET1/COMPASS complex and thus H3K4me3.
Based on these data, the authors proposed a model that helps explain the role of TET and OGT proteins in the epigenetic regulation of gene expression: TET2 and TET3 interact with OGT to promote GlycNAcylation of various proteins, including HCF1, and HCF1 GlycNAcylation is important for SET1/COMPASS complex formation. The SET1/COMPASS complex binds to chromatin to increase levels of the transcription-friendly H3K4me3 epigenetic mark and transcriptional activity of nearby gene promoters.
Thus, TET proteins play two roles in epigenetic gene regulation: They can convert 5-methylcytosine to 5-hydroxymethylcytosine, which seems to be involved in DNA demethylation and transcriptional activation, and increase the levels of H3K4me3 through their interactions with OGT and components of the SET1/COMPASS complex.
Of course, in the interest of space, I’ve left out a lot of the details. If you want to read more about the authors’ findings or learn more about their experimental design, check out the EMBO Journal article.
References
- Deplus, R. et al. (2013) TET2 and TET3 regulate GlcNAcylation and H3K4 methylation through OGT and SET1/COMPASS. EMBO J. 32, 645–55.
- Williams, K. et al. (2011) TET1 and hydroxymethylcytosine in transcription and DNA methylation fidelity. Nature 473, 343–48.
- Wu, H. et al. (2011) Dual functions of Tet1 in transcriptional regulation in mouse embryonic stem cells. Nature 473, 389–93.
- Ficz, G. et al. (2011) Dynamic regulation of 5-hydroxymethylcytosine in mouse ES cells and during differentiation. Nature 473, 398–402.
- Pastor, W.A. et al. (2011) Genome-wide mapping of 5-hydroxymethylcytosine in embryonic stem cells. Nature 473, 394–97.
- Gu, T.P. et al. (2011) The role of Tet3 DNA dioxygenase in epigenetic reprogramming by oocytes. Nature 477, 606–10.
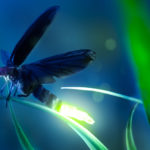
Terri Sundquist
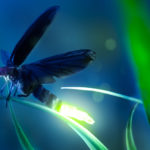
INTERESTING….!!!
Seems that epigenetics is now becoming as murky as the miRNA too….! The question i’ve been asking myself for about a decade is that, “has man come to his limit of his understanding,….or is it an educational ‘blackhole’ we reached…..!!!!
Happy weekend!
I’m afraid you might be right, Abduljalal. Epigenetics is “clear as mud”, at least for the foreseeable future. Fortunately, scientists are making progress in unraveling the mystery that is epigenetics. As with any scientific field of study though, the pace of discovery is frustratingly slow at times and there is much to learn.The important thing is that we are still moving forward and increasing our library of knowledge, and as long as scientists can do that, I don’t know that we’ll ever reach an “educational blackhole”.