From enzyme activity to gene expression, light-based assays have become foundational tools in life science research. Among these, fluorescence and bioluminescence are two of the most widely-used approaches for detecting and quantifying biological events. Both rely on the emission of light, but the mechanisms generating that light—and the practical implications for experimental design—are quite different.
Choosing between a fluorescence or bioluminescence assay isn’t as simple as picking between two reagents off the shelf. Each has strengths and limitations depending on the application, instrumentation, and biological system. In this blog, we’ll walk through how each method works, where they shine (and where they don’t), and what to consider when deciding which approach is right for your experiment.
How Light is Generated: Core Principles
Fluorescence
Fluorescence relies on an external light source—usually a laser or high-intensity lamp—to excite a fluorophore. When excited, the fluorophore absorbs light at one wavelength and emits it at a longer wavelength. Filters are used to separate the excitation and emission light, allowing the signal of interest to be detected.
This setup requires more complex instrumentation. Fluorescence readers or microscopes must include excitation and emission filters, optical components to manage light paths, and detectors that can distinguish between signal and background. These systems offer flexibility for multiplexing and imaging, but the hardware demands can add cost and complexity.
Fluorescence is highly versatile, but the need for external illumination also introduces challenges. Autofluorescence from cells or media and light scattering can interfere with detection, particularly when working with low-abundance targets or in heterogeneous samples.
Bioluminescence
Bioluminescence works differently. Instead of relying on an external light source, it generates light through a chemical reaction—typically involving an enzyme like luciferase and a substrate such as luciferin. When luciferase catalyzes the oxidation of luciferin, light is produced as a byproduct of the reaction.
Since this process doesn’t require excitation light, bioluminescent assays are less susceptible to background interference. Most biological systems don’t naturally emit bioluminescent light, so the signal-to-background ratio is inherently high. This makes bioluminescence especially valuable for detecting low-level signals in live cells or complex samples.
In terms of instrumentation, bioluminescent assays require a luminometer capable of detecting low levels of emitted light with high sensitivity. While these instruments don’t need the complex optics of fluorescence systems, sensitivity and dynamic range are critical for capturing accurate results.
Comparing Assay Sensitivity
One of the biggest differences between fluorescence and bioluminescence assays lies in their sensitivity—specifically, how well each method distinguishes signal from background noise.
Fluorescence assays can produce strong signals, especially with bright fluorophores or amplified detection strategies. However, high background levels can reduce effective sensitivity. Autofluorescence from cells, media components, or plasticware can interfere with detection, and over time, photobleaching can reduce the intensity of the signal—especially in live-cell or time-course experiments.
These factors make it harder to detect subtle changes or low-abundance targets, particularly in complex biological systems where background signal is hard to avoid.
Bioluminescent assays tend to have a much higher signal-to-noise ratio. Background is minimal by design, since the signal is generated enzymatically, and most biological systems lack endogenous bioluminescent activity. This makes bioluminescence an ideal choice for applications where sensitivity is key—such as tracking endogenous gene expression over time or monitoring signaling pathways in live cells.
Recent studies back this up. A 2023 review published in Biosensors (MDPI) highlights the advantages of bioluminescent systems for biosensor applications, noting that their low background and high dynamic range make them well-suited for detecting low-concentration analytes and real-time kinetic measurements1. Similarly, bioluminescent biosensors often outperform fluorescent counterparts in live-cell environments, particularly when weak signals needed to be distinguished from background noise2.
Applications and Use Cases
While both fluorescence and bioluminescence assays are widely used in biological research, their strengths make them better suited to different types of experiments. The choice often comes down to how much control you need over spatial resolution, timing, and sensitivity.
Fluorescence
Fluorescence-based methods are well-established across a broad range of applications. Their ability to provide spatial and temporal resolution makes them ideal for imaging experiments—from tracking labeled proteins in live cells to visualizing tissue architecture. Multiplexing is another major strength of fluorescence; with the right combination of fluorophores and filters, researchers can monitor multiple targets in the same sample.
These capabilities are essential for applications like flow cytometry and fluorescence microscopy, and advances in probe design and instrumentation continue to expand what’s possible. For example, fluorescence-based live-cell imaging enables researchers to visualize cellular events in real time. This Live Cell Imaging Resource Center offers a closer look at how these tools are applied across various biological systems.
Bioluminescence
Bioluminescence assays are well-suited for applications that require high sensitivity and minimal background interference. Because the signal is generated internally and doesn’t require external illumination, bioluminescence is a powerful choice for live-cell and kinetic assays, especially when low-abundance targets are involved.
Common uses include monitoring gene expression, measuring protein-protein interactions, and tracking dynamic signaling events over time. Bioluminescent assays also tend to be less phototoxic than fluorescence, making them gentler on live cells during longer experiments. For researchers looking to explore these applications further, this Luminescence Resource Center provides in-depth guides, protocols, and example data.
Live-Cell Imaging
Live-cell imaging gives researchers the ability to observe biological processes as they happen, rather than relying on fixed snapshots or endpoint measurements. This approach provides valuable data on dynamic cellular events like gene expression, protein localization and cell signaling all while preserving the native cellular environment. When combined with luminescent or fluorescent reporters, live-cell imaging enables both spatial and temporal resolution, making it possible to monitor changes over time and across different cellular compartments.
A key advantage of bioluminescence for imaging is the inherent stability and sustainability of the bioluminescent signal, which does not require external excitation like fluorescent tags. This allows direct visualization of protein dynamics in living cells without the need for repeated sample excitation. The lack of external excitation also reduces the risk of phototoxicity and photobleaching, common issues that can adversely affect cell viability and signal integrity over time.
Live-cell imaging offers a sensitive, low-background approach to monitor biological events in real time—ideal for applications where signal clarity and minimal disturbance to the cell are critical. Instruments like the GloMax® Galaxy Bioluminescence Imager—which integrate luminescence, fluorescence, and brightfield imaging in a single platform—support a range of live-cell studies. These include visualizing protein-protein interactions, monitoring protein degradation or target engagement, and tracking cell signaling dynamics—all in living systems where maintaining physiological conditions matters.
Practical Considerations and Choosing the Right Assay
While tools like the GloMax® Galaxy instrument support a range of applications, selecting the right detection method depends on several practical factors, from signal strength to instrumentation. There’s no universal winner when it comes to light-based assays—each method brings unique strengths, and the best choice depends on your specific experimental needs.
If sensitivity and low background are critical for your research—for example, when detecting weak signals in live cells or measuring dynamic responses over time—bioluminescence is often the better fit. The absence of autofluorescence and photobleaching makes it especially effective for low-abundance targets and long-term kinetic studies.
On the other hand, fluorescence remains the go-to for experiments that require multiplexing or high spatial resolution. Applications like flow cytometry and live-cell imaging often rely on the versatility and visual power of fluorescent probes.
Instrumentation is another consideration. While we’ve already touched on the complexity of fluorescence systems versus the sensitivity demands of bioluminescence readers, it’s worth noting that platform compatibility, throughput requirements, and lab workflow can also influence the decision.
To help compare options at a glance, the table below highlights key features of each assay type:
Feature | Fluorescence | Bioluminescence |
Signal Source | External excitation light | Enzymatic reaction (luciferase + substrate) |
Background Signal | Moderate to high (autofluorescence, scatter) | Low |
Sensitivity | Moderate to high | High |
Photobleaching | Can occur | Not applicable |
Multiplexing Capability? | Yes | Yes (Limited) |
Instrumentation Requirements | Filters, excitation source | Luminometer |
Common Applications | Imaging, flow cytometry, multiplex assays | Reporter assays, live-cell kinetics, low-abundance targets |
Conclusion
Both fluorescence and bioluminescence assays offer powerful ways to study biological systems, but they work best in different contexts. Fluorescence assays are suitable in imaging and multiplexing applications where spatial resolution matters, while bioluminescence assays stand out for their sensitivity and clean signal, especially in live-cell or low-abundance experiments.
Understanding how each assay works—and what trade-offs come with it—can help you design experiments that deliver clearer results and more confident conclusions. Whether you’re visualizing cells under a microscope or measuring gene expression in real time, the right detection method can make all the difference.
A Flexible Platform for Visualizing Assays
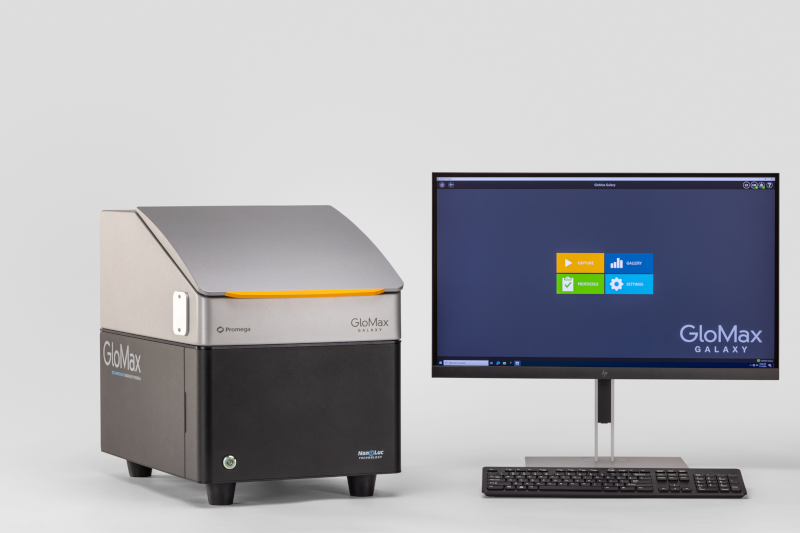
The GloMax® Galaxy Bioluminescence Imager is fully equipped for lluminescence, fluorescence, and brightfield imaging—ideal for visualizing NanoLuc® Luciferase-based assays like HiBiT, NanoBiT, and NanoBRET in both live-cell and end-point formats. Explore protein localization, degradation and interactions in real time—adding depth to your assays. See Your Research in a New Light With the GloMax® Galaxy.
The Glomax® Galaxy Instrument is for Research Use Only.
References
- Baader, W.J. et al. (2023). Biosensors, 13(4), 452. https://www.mdpi.com/2079-6374/13/4/452 ↩︎
- Matson, R.S. (2023). Methods in Molecular Biology. https://pubmed.ncbi.nlm.nih.gov/36795370/ ↩︎
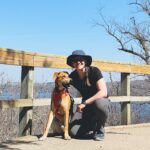
Anna Bennett
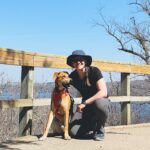
Latest posts by Anna Bennett (see all)
- Bioluminescence vs. Fluorescence: Choosing the Right Assay for Your Experiment - April 24, 2025
- No More Dead Ends: Improving Legionella Testing with Viability qPCR - March 18, 2025
- Strengthening Water Safety Measures with Advanced Detection - February 18, 2025