Introduction
RNA molecules have become a hot topic of research. While I was taught about messenger RNA (mRNA), ribosomal RNA (rRNA) and transfer RNA (tRNA), many more varieties have come into the nomenclature after I graduated with my science degrees. Even more interesting, these RNAs do not code for a protein, but instead have a role in regulating gene expression. From long non-coding RNA (lncRNA) to short interfering RNA (siRNA), microRNA (miRNA) and small nucleolar RNA (snoRNA), these classes of RNAs affect protein translation, whether by hindering ribosomal binding, targeting mRNA for degradation or even modifying DNA (e.g., methylation). This post will cover the topic of microRNAs, explaining what they are, how researchers understand their function and role in metabolism, cancer and cardiovascular disease, and some of the challenges in miRNA research.
What are microRNAs? MicroRNAs (miRNAs) are short noncoding RNAs 19–25 nucleotides long that play a role in protein expression by regulating translation initiation and degrading mRNA. miRNAs are coded as genes in DNA and transcribed by RNA polymerase as a primary transcript (pri-miRNA) that is hundreds or thousands of nucleotides long. After processing with a double-stranded RNA-specific nuclease, a 70–100 nucleotide hairpin RNA precursor (pre-miRNA) is generated and transported from the nucleus into the cytoplasm. Once in the cytoplasm, the pre-miRNA is cleaved into an 18- to 24-nucleotide duplex by ribonuclease III (Dicer). This cleaved duplex associates with the RNA-induced silencing complex (RISC), and one strand of the miRNA duplex remains with RISC to become the mature miRNA.
How do miRNAs affect gene expression?
The mature miRNA has a seed region of 6–9 bases near the 5´ end that defines its target. miRNAs direct the RISC complex to the 3´ untranslated region (UTR) of target mRNAs that may either block ribosomes from translating mRNA, recruit translation proteins or induce mRNA degradation. An individual miRNA has only a modest effect on gene expression, finely adjusting protein translation. However, the 6–9 base seed region means an individual miRNA may target several mRNAs, and that many different miRNAs can recognize and bind to an individual transcript. Having multiple miRNAs binding to a single transcript has a cumulative effect, thus downregulating gene expression.
In addition, miRNAs have been found in the nucleus, binding to promoters and regulating transcription of genes. miRNAs also can be found in exosomes, plasma membrane-based vesicles that bud from cells, and once secreted into the extracellular environment, can be delivered to other cells or interact with cellular receptors and trigger a response.
Why are researchers interested in miRNAs?
Since the discovery of miRNAs over two decades ago, researchers have been trying to understand miRNA function in plants and animals and were intrigued by the vast influence these small molecules have over many cellular systems. In fact, a few hundred conserved miRNAs are estimated to control 30–80% of human genes, an impressive pool of RNA regulatory molecules to investigate. Consequently, miRNAs regulate important systems including energy metabolism, cellular growth and differentiation. Researchers have found that miRNAs have tissue-specific expression and show concentration-based effects in some disease states. In addition, miRNAs are stable enough to be found in blood and are a promising area of research as biomarkers, potential indicators of pathway dysregulation in a less invasive manner and possibly even at an earlier time point compared to conventional methods. Biomarkers that are able to give real-time feedback about the efficacy of a possible drug treatment are also in great demand, and miRNA research suggests the blood stability of miRNAs could prove key to profiling cellular changes over time. Because of their association with human disease states, miRNAs are also potential targets for screening test compounds for drug development. Furthermore, new miRNAs are still being discovered with 2,588 mature miRNAs listed in miRBase.
What roles do miRNAs play?
Cancer research has been a large focus of miRNA research. When comparing a normal tissue sample with a cancerous one derived from the same tissue type, miRNA expression is significantly different. Research has demonstrated that miRNAs can act as oncogenes or tumor suppressors. miR-21 and miR-155 are frequently overexpressed in human cancer. The miR-15a/16-1 gene cluster acts as tumor suppressors because these miRNAs are often deleted in chronic lymphocytic leukemia (CLL). In some cases, a single miRNA may be both oncogene and tumor suppressor, depending on the tissue in which it is being expressed. miR-122 is overexpressed in liver cancers and targets PTEN, a tumor suppressor. But in other tumors, miR-122 is downregulated so expression of target oncogenes are no longer repressed.
In addition, miRNAs that exit the cells as free molecules or in vesicles can be delivered to target cells, acting as hormones that alter a tumor state. For example, one study showed miR-210 released from breast tumor cells via exosomes promoted angiogenesis and metastasis in endothelial cells. Like other gene alterations that are commonly found in cancers, miRNAs allow tumor cells to evade apoptosis, replicate without limit, evade immune destruction and demonstrate other activities associated with cancer. In lymph node metastases of lung cancer, miR-31 was identified as upregulated. In cell culture lung adenocarcinoma cells, miR-31stimulated the ERK1/2, a known oncogenic signaling pathway.
How these changes in miRNA expression occur are still under investigation, but they result in abnormal levels of mature or precursor miRNA or both. Many miRNA coding regions are located in introns and sometimes in close association with the genes they regulate. Therefore, if a particular cancer may delete or amplify that gene, the miRNA is affected as well. Methylation of miRNA genes (epigenetic regulation) and alterations to the miRNA processing genes or proteins can change miRNA expression and initiate or promote a cancer.
While many miRNAs are studied individually, researchers have learned using a panel of miRNAs known to be altered in cancers is better at classification and discrimination than examining a single miRNA. In addition, research also suggests some miRNAs may be able to classify a cancer as a higher or lower risk as well as indicate the possibility of responding to one treatment over another. Many of the current tests for cancer are invasive and expensive so the possibility of having blood drawn and miRNAs profiled for an early indication of cancer is a priority for cancer researchers. The use of miRNA in cancer research is evolving, but has great potential for future applications.
Metabolism is another research topic in which miRNAs play a role. Energy metabolism (e.g., glucose) involves several extensive signaling pathways. miRNAs target multiple genes and the network of genes that make up the signaling pathways. Not surprisingly, miRNAs affect glucose metabolism. miR-375 function was determined by cloning from murine pancreatic islets and learning that it downregulated glucose-stimulated insulin secretion. More miR-200 is found in islets from diabetic mice; more specifically, miR-200b targets Zeb1, resulting in apoptosis in pancreatic cells. The commonality among the miRNA clusters uncovered in this research is that only under cellular stress do these miRNAs show a response.
miRNAs are also involved in lipid and cholesterol metabolism. Of all the miRNAs expressed in the liver, ~5% is miR-122. When miR-122 expression is inhibited, lipid and cholesterol profiles improve in both wild-type and diet-induced obesity mice. miR-26 is decreased in the livers of individuals who are overweight and when expressed normally, protects against insulin resistance.
If miRNAs are involved in glucose metabolism, they are likely to play a role in insulin signaling pathways as well. miRNAs in the let-7 family are known to regulate glucose metabolism in the pancreas, muscle and liver. If let-7 is overexpressed in the pancreas or skeletal muscle of mice, the miRNA increases insulin resistance and reduces glucose tolerance. Transgenic mice that overexpress miR-143 decrease their insulin sensitivity. In all these instances, metabolic stress changes miRNA expression and results in reduced insulin sensitivity and dysregulated glucose metabolism.
Being involved in glucose metabolism and insulin signaling means researchers have shown numerous miRNAs are associated with obesity and type 2 diabetes in humans. In adipose tissue, miRNAs also influence adipocyte differentiation. miR-143 and miR-103/107 promote insulin resistance by suppressing white adipocyte differentiation.
While researchers know that miRNAs exert effects on metabolism, only a small number have been identified. There are likely many more that affect lipid and glucose metabolism and have a role in obesity and diabetes. Not only are there likely more miRNAs to be discovered but more targets as well. Like for cancer research, having biomarkers for changes in glucose metabolism would be useful when investigating metabolism and its regulation and may even offer targets for drug screening.
Cardiovascular disease
As with cancer research, the potential for cardiovascular-specific biomarkers in blood are important to researchers. While there are blood-based protein biomarkers currently used in cardiovascular disease, the cardiac troponin assay does not differentiate coronary artery disease (CAD) from noncoronary cardiac pathologies, and acute myocardial infarction (AMI) does not release troponin immediately. Research suggests that miRNA could discriminate among different cardiac pathologies. Cardiac tissue analyzed between individuals who died from myocardial infarction (MI) and otherwise healthy individuals showed miR-208 was upregulated while miR-1 and miR-133a/b was downregulated in those with MI. miR-1 is important because it is an abundant miRNA that is specific for cardiac and skeletal tissue and regulates cardiomyocyte growth.
Atherosclerosis is another area of research focus and involves inflammation and cholesterol homeostasis. There are several miRNAs involved in angiogenesis and endothelial monolayer integrity. One mouse model showed miR-223 regulated cholesterol synthesis, uptake and efflux, suggesting a potential role for this miRNA in atherosclerosis. This correlates with the elevated levels of miR-223 found in thrombocytes of individuals with atherosclerosis.
Similar to cancer researchers, scientists investigated panels of miRNAs to discover if there was a signature associated with cardiovascular disease. In fact, researchers were able to distinguish healthy controls from individuals with CAD that had either unstable angina pectoris (UAP) or stable angina pectoris (SAP) using miRNAs. UAP was associated with elevated levels of miR-1, miR-126 and miR-133a and SAP with increased levels of miR-1, miR-126 and miR-485-3p.
Investigation into potential compounds for cardiovascular disease also target miRNAs. Researchers are exploring how to increase or decrease miRNA expression to achieve the desired results. Under consideration is miRNA mimic technology to help downregulate undesired proteins. Alternatively, an miRNA could be directly suppressed or even inactivate by mimicking the targets of an miRNA to block the miRNA activity.
What are the challenges in using miRNAs in research?
Currently, there are no standards for collecting cell or tissue samples for miRNA detection and purification. Because there are expression differences in various tissue types, using sample types like whole blood versus serum or plasma seems to show different results when detecting miRNAs and assaying expression levels. Even if researchers can collect the same tissue types (e.g., normal and cancerous breast tissue from a single source), there is no standard against which to assess miRNA expression levels. For example, qPCR analysis typically uses a housekeeping gene that is amplified along with the gene of interest to account for inherent sample variability. No such equivalent exists for miRNA.
Once the sample is collected, how to isolate the miRNA? There are plenty of protocols and even commercial kits available (e.g., the ReliaPrep™ miRNA Cell and Tissue Miniprep System) to extract miRNA, but there is no universally recommended protocol for use across all research. Some protocols result in enriched miRNA fractions and other extract total cellular RNA. In addition, there are several methods used to detect miRNA, but no universal guidelines on which method to use for a particular sample type or when wanting to assay a particular miRNA or set of miRNAs. Assessing miRNA concentration and integrity is even more challenging than mRNA molecules due to their small size, but both qualities affect the results in downstream assays.
Conclusion
miRNA is a still evolving field of research and there is much unknown about the noncoding regulatory RNA molecules. What has been revealed, the roles played in cancer, metabolism and cardiovascular health, shows a class of molecules that have multifactorial effects. miRNAs fine tune gene expression and any changes in their expression have wide-reaching affects. While the RNA molecules may be less than 30 nucleotides long and use a target of 6–9 bases, miRNAs have several cellular mRNA and even DNA targets upon which to act. Current research offers hints that miRNAs could be future biomarkers for disease states and offer more insight into disease development. In addition, miRNAs may be targets of future screening efforts for drug development. Despite over twenty years of research, there is still much to learn about miRNAs.
References
- Berindan-Neagoe, I., Monroig Pdel, C., Pasculli, B. and Calin, G.A. (2014) MicroRNAome genome: a treasure for cancer diagnosis and therapy. C.A. Cancer J. Clin. 64, 311–36. doi: 10.3322/caac.21244
- Hartig, S.M., Hamilton, M.P., Bader, D.A., McGuire, S.E. (2015) The miRNA interactome in metabolic homeostasis. Trends Endocrinol. Metab. 26, 733–45. doi: 10.1016/j.tem.2015.09.006
- Schulte, C. and Zeller, T. (2015) microRNA-based diagnostics and therapy in cardiovascular disease-Summing up the facts. Cardiovasc. Diagn. Ther. 5, 17–36. doi: 10.3978/j.issn.2223-3652.2014.12.03
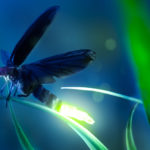
Sara Klink
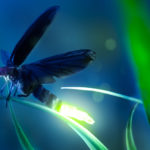
Latest posts by Sara Klink (see all)
- A One-Two Punch to Knock Out HIV - September 28, 2021
- Toxicity Studies in Organoid Models: Developing an Alternative to Animal Testing - June 10, 2021
- Herd Immunity: What the Flock Are You Talking About? - May 10, 2021
A pretty nice piece!
Keep it Up!